•A Report of the CSIS Missile Defense Project
By Tom Karako, Masao Dahlgren
DOWNLOAD THE REPORT
WATCH THE EVENT
Summary
Hypersonic weapons combine the speed of ballistic missiles with the maneuverability and detectability challenges of cruise missiles, leaving little time to react. In the past five years, Russia, China, and others have accelerated their development of hypersonic missiles to threaten U.S. forces in the homeland and abroad. The current Ballistic Missile Defense System, largely equipped to contend with legacy ballistic missile threats, must be adapted to this challenge. The same characteristics that make hypersonic missiles attractive may also hold the key to defeating them. A new hypersonic defense architecture should exploit hypersonic weapons’ unique vulnerabilities and employ new capabilities, such as a space sensor layer, to secure critical nodes. These changes are not only necessary to mitigate the hypersonic threat but to defeat an emerging generation of maneuvering missiles and aerial threats.
Introduction
Defending against hypersonic missiles is strategically necessary, technologically possible, and fiscally affordable, but it will not be easy. Its realization will require different approaches and new ways of
thinking from those employed for legacy ballistic and cruise missile defense missions. Hypersonic weapons combine the speed and range of ballistic missiles with the low-altitude and maneuverable
flight profile of a cruise missile. While traditional defenses can handle these challenges individually, their combination will require new capabilities, operational concepts, and defense design. The same
characteristics that make hypersonic missiles attractive may also hold the key to defeating them. Instead of thinking about hypersonic defense as an adjunct to the legacy ballistic missile defense problem, it might be better understood as a form of complex air defense.
Decades ago, the United States put the world on notice of its intent to field ballistic missile defenses with a particular focus on intercepting predictable targets outside the atmosphere. Since then, the missile threat spectrum has broadened, becoming more endoatmospheric and more maneuverable.
In the 2000s, Russia and China developed a variety of unmanned aerial systems, advanced cruise missiles, hypersonic glide weapons, and increasingly sophisticated ballistic missiles. The characteristics
of these weapons reduce defensive system coverage, force defenders to look multiple directions at once, and require more agile interceptors.
Since 2018, Russia has tested at least four new hypersonic or high-speed weapons, inducting into service its long-range Avangard hypersonic glide vehicle and Kinzhal air-launched ballistic missile. China, meanwhile, has tested or fielded several types of hypersonic weapons and has outpaced the United States in graduating hypersonic-specialized engineers, publishing open scientific papers, and constructing hypersonic wind tunnels.1Chinese and Russian investments in advanced missile capabilities are part of what the March 2021 Interim National Security Strategic Guidance identified as “efforts meant to check U.S. strengths and prevent us from defending our interests and allies around the world.”2
Hypersonic defense is necessary to disrupt these efforts, defend U.S. territory, and protect forward deployed forces and the defense posture they support.Effective deterrence leverages both the threat of
punishment and a credible capability to deny an attack. A mix of active and passive defense measures will raise the threshold for aggression, increase uncertainty in an adversary’s decision calculus, and impose developmental costs on adversaries. Effective defensive capabilities buy time to bring other military, diplomatic, and economic responses to bear and mitigate policy and operational challenges associated with attacking missile launchers in a crisis. Developing hypersonic defenses need not and should not happen in a vacuum or as a new standalone stovepipe. Hypersonic defense can leverage ongoing investments in both ballistic and cruise missile defenses and hypersonic strike, all of which draw upon a similar industrial base and leverage similar sensors and networks.
Senior Biden administration officials have affirmed the importance of hypersonic defense. During his confirmation process to become secretary of defense, Lloyd Austin stated that he would “encourage
efforts to address the full spectrum of missile threats, including . . . the accelerated development of intercept capability for hypersonic missile defense.”3 Deputy Secretary Kathleen Hicks likewise pledged a focus on the necessary sensor capabilities: “If confirmed, I would assess ongoing efforts to improve national missile defense, with a particular focus on improving discrimination capabilities and sensors
for detection of both ballistic and hypersonic missiles.”4
The characteristics of hypersonic missiles may seem novel, but they are in fact the harbinger of a new age of missile warfare.5New ballistic missiles are being flown with lower and more heavily shaped
trajectories. New cruise missiles sustain higher speeds and are becoming more difficult to detect. Future threats will include missile-drone combinations, spaceplanes, aeroballistic missiles, and other hybrids that strain simple categorization. Hypersonic missiles thus do not represent a boutique problem. They exemplify a broader evolution in the missile threat—one which demands changes to the broader missile defense paradigm.
The 2019 Missile Defense Review noted that “the scale and urgency of change required to restore conventional and missile defense overmatch should not be underestimated.”6The scale and urgency
required here touches virtually every aspect of missile defense: sensors, interceptors, defense design, doctrine, and policy. The problem of complex air and missile defense is not about some still-emerging future threat. It is a present imperative to contend with hypersonic weapons already deployed and ready for use, and others soon to come. U.S. defense officials have long expressed the need to convert the major defense acquisition program, called the Ballistic Missile Defense System, into a Missile
Defense System to contend with a threat spectrum no longer defined by ballistic missiles. Now the time has come to do so.
Findings
▪Fielding hypersonic defenses will require an integrated, layered, system-of-systems approach, new sensing and interceptor capabilities, different operational concepts, doctrinal and organizational changes, and modified policy expectations.
▪ Hypersonic flight is by definition atmospheric flight. As such, hypersonic defense might be better understood as a complex form of air defense rather than as an adjunct to ballistic missile defense.
▪ Hypersonic missile threats should be a key driver for rethinking the approach to missile defense and defeat as well as for the emergence of the Missile Defense System out of the Ballistic Missile Defense System. Doing so will benefit the development of defenses against other non-ballistic threats, including subsonic and supersonic cruise missiles, loitering munitions, and other novel delivery systems.
▪ The single most important program element for hypersonic defense is a resilient and persistent space sensor layer capable of observing, classifying, and tracking missile threats of all types, azimuths, and trajectories.
▪ The second most important program element is a glide-phase interceptor. Thus far, hypersonic defense investments have been modest, just a small fraction of that devoted to hypersonic strike. At the current pace, a glide-phase interceptor may not be fielded until the 2030s, but this timeline
could be accelerated.
▪ Even without a space sensor layer and a glide-phase interceptor, a defense design can employ existing sensors and alternative effectors in ways that constrain a hypersonic missile’s maneuver budget, channel the threat, and impose other costs in ways favorable to the defender.
▪ The same characteristics that make hypersonic weapons attractive present the defender with new failure modes to exploit. A comprehensive approach may benefit by supplementing hit-tokill
intercept with area-wide effectors, including high-powered microwave systems, twenty-first century versions of flak, and other means to target vulnerabilities of the hypersonic flight regime.
▪ The United States does not compete with unlimited resources. It is not possible to actively defend every critical asset or even broad areas that hypersonic missiles might target. This simple reality requires policy and strategy expectations aligned to preferential defense and a more limited
defended asset list. Regional and force protection missions, and a small number of critical assets in the homeland, should be prioritized.
▪ Current doctrinal and organizational constructs impede information sharing, communication, and decisionmaking. These require adaptation to support counter-hypersonic operations across
multiple domains, commands, and areas of responsibility.
▪ Hypersonic defense efforts require the United States to regain related scientific and industrial leadership through the maintenance of predictable budgets, deepened cooperation with allies,
investments to remove industrial bottleneck areas, improved testing and modeling infrastructure, and sustained continuity of effort.
▪ The challenge of defending against the full spectrum of hypersonic missile threats will not be met by a single, silver-bullet solution. Numerous efforts tailored to exploit key vulnerabilities of hypersonic flight can make hypersonic defense a more tractable problem.
An Attribute, Not a Thing
▪ Beginning around Mach 5, flying objects encounter thermal and aerodynamic phenomena distinct from those encountered in supersonic and exoatmospheric flight. These phenomena define the hypersonic flight regime.
▪ The combined characteristics of high speed, lower altitude, and maneuverability make it difficult to predict the trajectories of hypersonic weapons, especially with terrestrial sensors. Although ballistic missiles, cruise missiles, and certain aircraft share some of these characteristics, their combination presents a qualitatively different problem.
▪ While hypersonic weapons are normally divided into two categories—hypersonic glide vehicles and hypersonic cruise missiles—this bifurcation does not reflect the actual and potential diversity of the hypersonic missile threat spectrum.
▪ Hypersonic flight is by definition atmospheric flight. As such, hypersonic defense might be better understood as a complex form of air defense.
▪ The characteristic challenges of hypersonic flight raise intriguing possibilities for a defender.
Hypersonic weapons are those that travel over Mach 5, or five times the speed of sound. But speed is not what differentiates hypersonic weapons from other missiles. Long-range ballistic missiles and orbital bombardment systems reach similar or greater speeds as they reenter the atmosphere. What makes hypersonic weapons attractive is rather their ability to sustain these speeds at altitudes
below those of most ballistic missiles, and above all to maneuver while doing so. The altitude range for hypersonic flight is below 100 km, where space is often said to begin, and typically around 20 to 60 km, above the ceilings of most aircraft and cruise missiles.7 The combined characteristics of high speed, lower altitude, and maneuverability stress existing defenses.
Figure 1: Hypersonic and Ballistic Trajectory Comparison
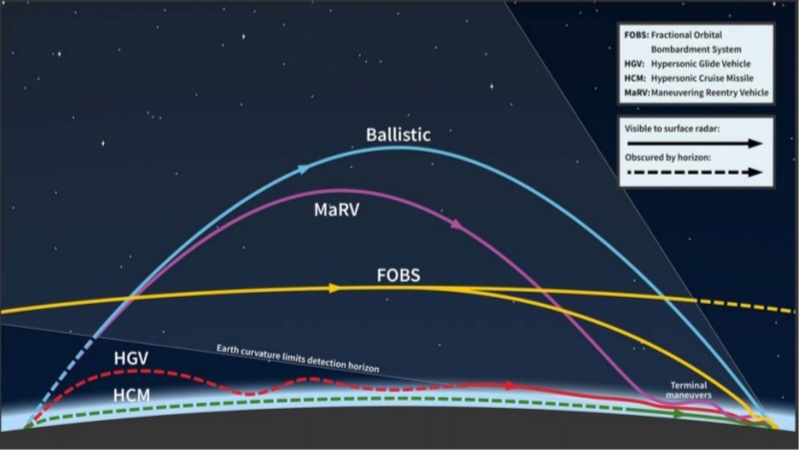
Source: CSIS Missile Defense Project. Trajectories not to scale.
Ballistic reentry vehicles travel at high velocities in the vacuum of space and thus briefly experience hypersonic conditions upon reentry. Maneuvering reentry vehicles and spaceplanes are also cousins to
hypersonic weapons. But none of these other forms experience the aerothermal stresses of extended hypersonic flight.
Hypersonic flight is better understood as the regime where certain aerodynamic and thermal phenomena that are not common to subsonic or lower supersonic flight begin to occur. These phenomena involve
extreme temperatures and aerothermal interactions on the vehicle surface. Of particular importance are the remarkable amounts of flow friction and viscous dissipation encountered by the hypersonic vehicle, leading to substantial temperature increases, the dissociation and ionization of surrounding gases, and the formation
of plasmas. Hypersonic weapons must be capable of surviving this environment for a sustained period.
Figure 2: Atmospheric Speed Regimes
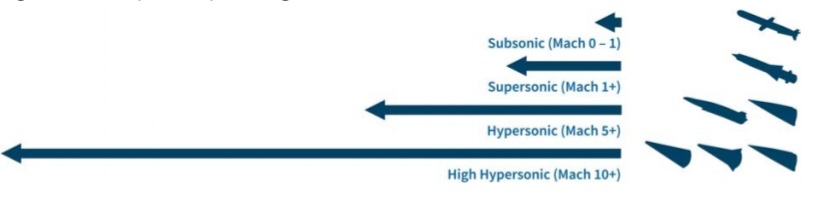
Source: CSIS Missile Defense Project.
Figure 3: Mach Number True Speeds by Altitude
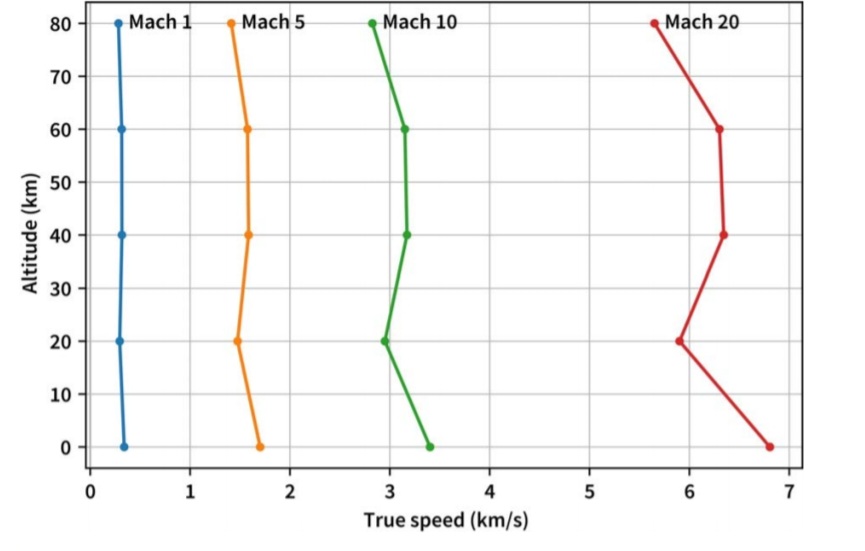
The onset of hypersonic conditions may vary according to several factors.
Source: CSIS Missile Defense Project, with data from National Oceanic and Atmospheric Administration.
This set of physical phenomena, not a specific speed or Mach number, defines hypersonic flight. Objects traveling at similar speeds in space do not encounter hypersonic conditions until they reenter
the atmosphere. Just as Mach numbers only make sense in reference to atmospheric conditions, it does not make sense to describe an object as hypersonic if it is in the vacuum of space (Figure 3).
Furthermore, reaching the hypersonic flight regime is not like flipping a light switch. As with certain characteristics of transonic flight, the range between about Mach 0.8 and 1.2, the phenomena of the hypersonic flight regime develop and change over a spectrum.
“Hypersonic really is a class of supersonic flight that is characterized by pushing the
envelope relative to the . . .aerothermodynamic performance of a vehicle. . . . People think of hypersonics as a thing: ‘I’m going to go buy a hypersonic.’ And that means I’ve got one thing that’s ‘a hypersonic.’ It really is not a thing, it’s an attribute. It’s a capability.”8
— Michael White, Office of the Under Secretary of Defense for Research and Engineering
Just as the word “ballistic” describes a parabolic trajectory defined by gravity, the word “hypersonic” refers to a quality of high supersonic flight at which these phenomena occur. Neither “ballistic” nor
“hypersonic” defines a particularpropulsion type or kind of missile. The use of “hypersonics” as a noun does not designate a missile or aircraft type but rather a field of study, such as those of ballistics and electronics. In the words of Michael White, principal director for hypersonics within the Office of the Under Secretary of Defense for Research and Engineering, one does not acquire “a hypersonic” but instead a missile that may for some period of its trajectory happen to travel at hypersonic speeds. “Hypersonic” is an attribute, not a thing—an adjective, not a noun.
“Just as Mach numbers only make sense in reference to atmospheric conditions, it does not make sense to describe
an object as hypersonic if it is in the vacuum of space.”
Taxonomy Blurring into Spectrum
Hypersonic missiles are typically categorized by their source of propulsion: rocket-boosted gliders and air-breathing cruise missiles. While a hypersonic cruise missile (HCM) uses an onboard propulsion
system to reach hypersonic speeds, a hypersonic glide vehicle (HGV)—also called a boost-glide vehicle—is unpowered, entering the hypersonic regime after an initial acceleration from a detachable
booster. Like a ballistic reentry vehicle, an HGV typically boosts into space or near-space at the beginning of flight using a rocket motor. Instead of continuing along a ballistic trajectory, however, an HGV detaches and reenters the atmosphere at high speeds. With a shape optimized to reduce drag and produce lift, an HGV can then glide through the atmosphere using its inherited momentum.9 Some HGVs use “skipglide” maneuvers to increase range, skipping like a stone on the density gradient between space and the upper atmosphere. In general, unpowered HGVs gradually lose speed during their glide phase, slowing to lower hypersonic (Mach 5 to 7) or supersonic speeds.
An HCM, by contrast, typically uses an airbreathing supersonic-combustion ramjet, or scramjet, instead of rocket-powered thrust. Like other cruise missiles, HCMs remain under powered flight through most of their trajectory and often cruise at lower hypersonic speeds and altitudes than HGVs.10 While HCMs present weight, packaging, and efficiency advantages over HGVs, scramjet engines’ sensitivity to airflow disturbances
often limits their ability to perform extremely sharp maneuvers.11
Figure 4: Relation of Weapon Categories
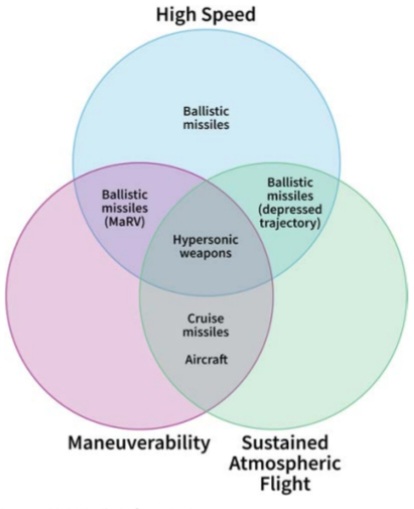
Source: CSIS Missile Defense Project.
The common HGV/HCM bifurcation oversimplifies the spectrum of hypersonic missile design possibilities. Focusing on these two types alone impedes anticipating future threats. Future hypersonic systems may, for instance, employ a combination of these propulsion methods or another altogether. A scramjet or other device could be integrated into a glider to increase range or maneuverability.12 Spaceplanes and fractional or multiple orbital bombardment systems further escape the HGV/HCM
dichotomy. In July 2021, China reportedly orbited an object that, after circling the earth, deorbited and then executed hypersonic flight maneuvers during atmospheric reentry.13 Hypersonic airframes of the future may be reusable platforms as well as projectiles and may serve as payload trucks to service
multiple missions. Numerous such concepts have been in circulation since the dawn of the missile age and may yet come to fruition.
Figure 5: Historical Hypersonic Glide Body Research
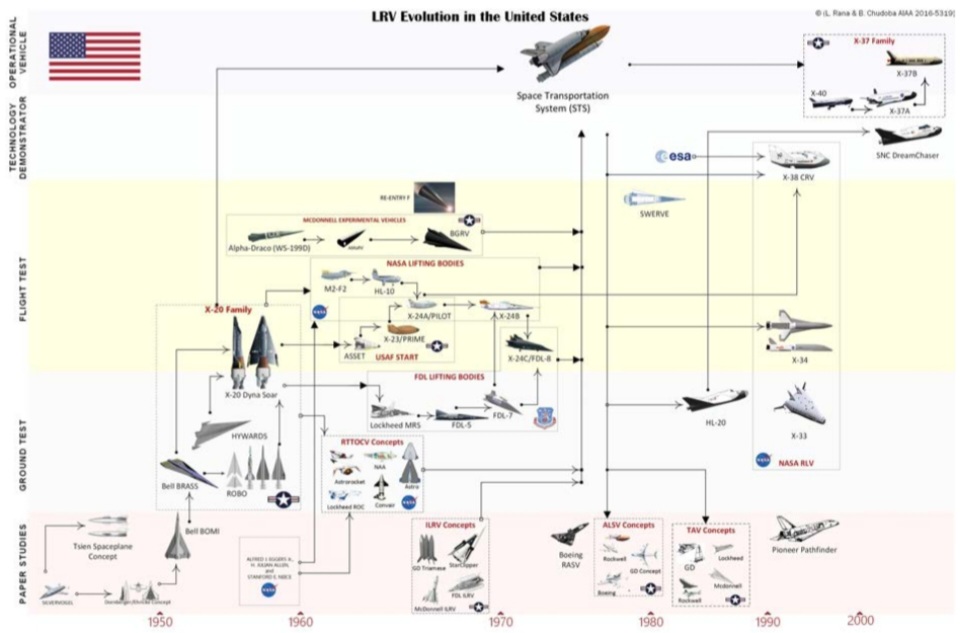
Source: Loveneesh Rana, University of Luxembourg, and Bernd Chudoba, University of Texas, Arlington. Reprinted with permission.
While not designed to survive extended flight in the hypersonic regime, maneuvering reentry vehicles (MaRVs) are close cousins of HGVs. MaRVs may also pull high-G turns at hypersonic speeds even though they do not sustain hypersonic flight or possess the same aerodynamic lift characteristics as HGVs. Despite such differences, much of the basis for contemporary U.S. hypersonic glide weapons emerged out of research for MaRVs.14 Several varieties of ballistic missiles spend significant portions
of flight in the atmosphere and can perform limited midcourse and terminal maneuvers. Due to these characteristics, some so-called “aeroballistic” or “quasi-ballistic” missiles are occasionally misdescribed as hypersonic weapons (Figure 6).
Figure 6: Weapon Characteristics and Commonalities
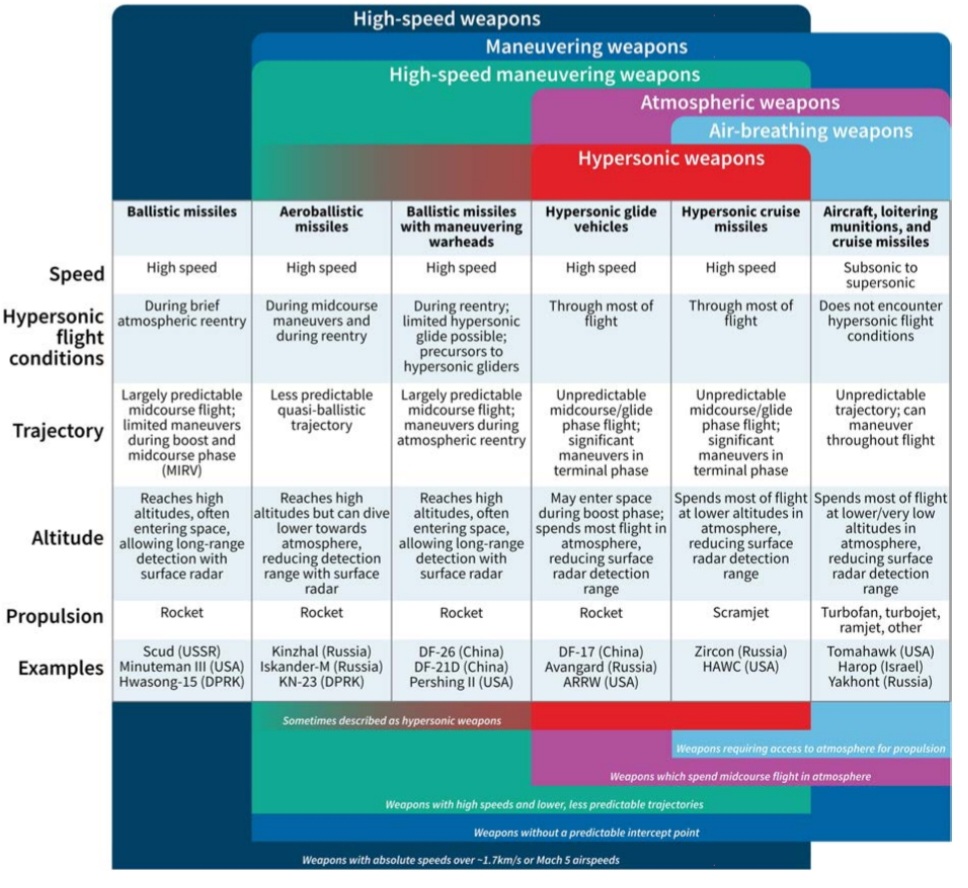
Source: CSIS Missile Defense Project.
The attributes of hypersonic missiles represent a broader trend in advanced missilery away from purely ballistic trajectories and toward lower, more maneuverable, more aerodynamic, and less predictable flight.15 Drawing attention to the scope of characteristics and trends, rather than a specific weapon or type, will be critical to anticipate the breadth of future defense needs.
Vulnerabilities of Hypersonic Flight
Some of the same characteristics that make advanced hypersonic missiles desirable present opportunities that defenders could seek to exploit. The physical phenomena of sustained hypersonic flight offer vulnerabilities that should inform hypersonic defense design. Hypersonic flight is by definition atmospheric flight. As such, hypersonic defense might be better understood as a complex form of air defense. Defining it as such is necessary to anticipate and contend with the increasingly diverse spectrum of threats “arriving in or through the atmosphere.”16
Hypersonic weapons experience challenging aerothermal conditions that strain the limits of current guidance, control, and materials technologies. After reentering the atmosphere at speeds of around Mach 20, an intercontinental-range hypersonic glider experiences extreme pressures and vibration modes, as well as temperatures topping 4,000 degrees Fahrenheit.17 In such an environment, the vehicle’s surrounding atmosphere dissociates into a plasma, reacting violently with the airframe’s surface.
Whereas ballistic reentry vehicles experience similar conditions for tens of seconds during atmospheric reentry, hypersonic weapons must survive similar conditions for many minutes (Figure 7).18
Figure 7: Depicting Hypersonic Flow Phenomena
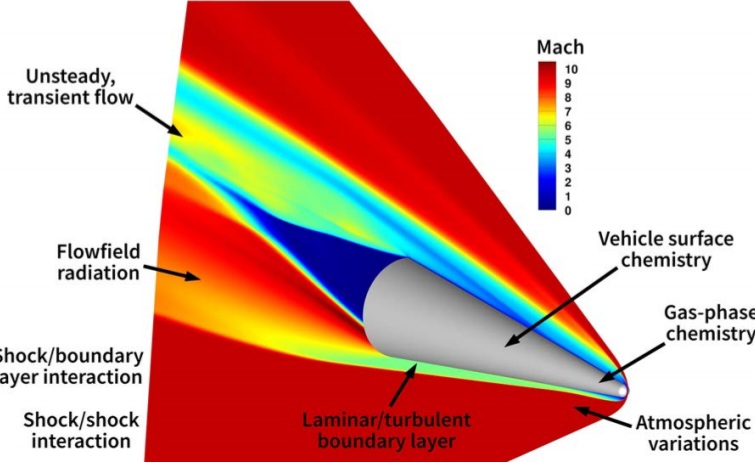
Note: Shock/shock interaction and shock/boundary layer interaction not depicted.
Source: Reprinted with permission from Justin Smith, Sandia National Laboratories.
Ensuring reliable performance in this environment often requires exotic materials and highly integrated designs, especially for higher speeds.19 While commercial aircraft subsystems can often
be designed separately and integrated later, changes to a hypersonic airframe’s structure, avionics, or heat shield materials can alter design considerations for every other subsystem.20 Minor alterations
in the basic shape or weight distribution in a hypersonic vehicle’s airframe, for instance, can have downstream effects on thermal and propulsion system performance and on accuracy.21 Designs chosen for lift, drag, or stability characteristics may require the selection of heavier insulation or differently
shaped inlets, which in turn feed back into vehicle shape, cost, mass, and control system decisions.22
“Changes to a hypersonic airframe’s structure, avionics, or heat shield materials can alter design considerations for every other subsystem.”
These problems are compounded by the difficulty of predicting hypersonic system performance. Various physical phenomena interact in nonlinear ways to heat and destabilize a hypersonic vehicle or disrupt scramjet engine performance (Figure 9).23 Difficulties in modeling boundary layer transition—the sudden formation of hot, turbulent airflow around a vehicle—stymied earlier efforts to master hypersonic flight (Figure 8).24 Predicting boundary layer transition requires designers to model a larger number of variables, including vehicle shape and the surrounding airflow, surface texture, and—at high hypersonic speeds and within hypersonic engines—chemical interactions between superheated gas plasma and the vehicle surface, which themselves change over time as the surface erodes.25 An
inability to accurately predict these challenges may force designers to employ additional margins of thermal protection, increasing vehicle size and weight.26 These and other factors translate to problems with vehicle stability and control. Hypersonic systems are difficult to design and operate in part because the variables that govern their performance are closely coupled.
Figure 8: Laminar and Turbulent Boundary Layers
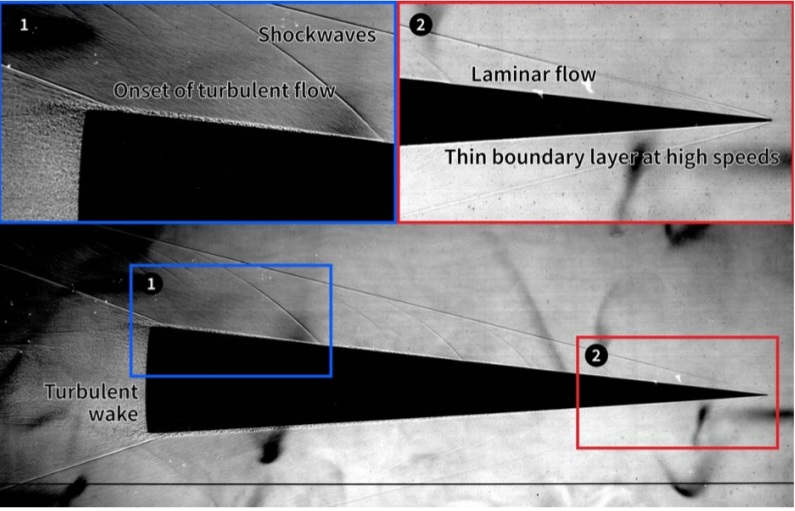
Shadowgraph of Mach 4 cone, taken at Naval Ordnance Laboratory Ballistics Range, 1979.
Source: Daniel C. Reda, Naval Surface Weapons Center. Edited by CSIS Missile Defense Project. Reprinted with permission.
Figure 9: Select Hypersonic Flow Phenomena27
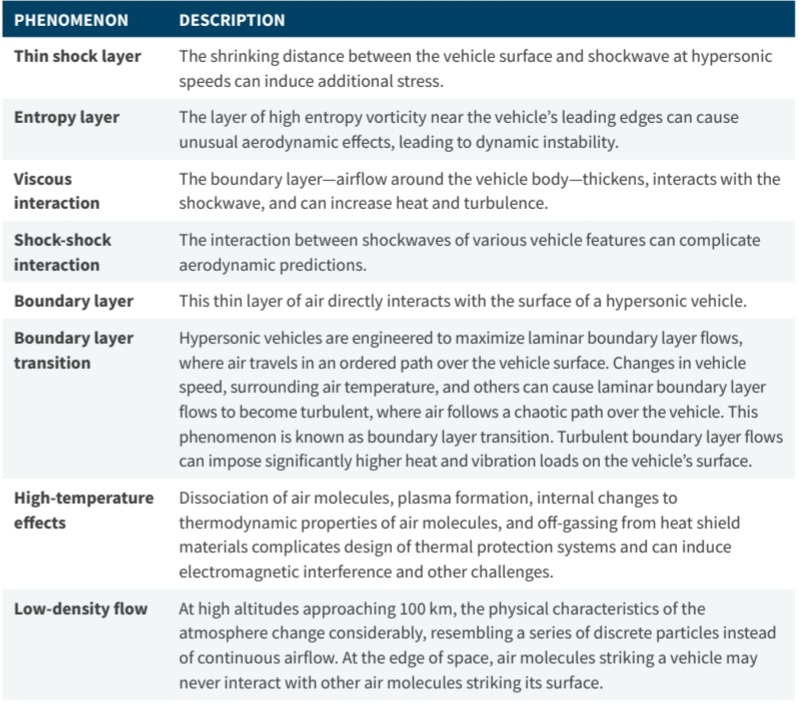
Defense Is Possible
Hypersonic missile defense is possible. Realizing it, however, requires rethinking existing defense designs and a willingness to approach the problem differently. Targeting the specific characteristics of
hypersonic flight can break the problem into manageable pieces, favorably affect the offense-defense cost curve, and make the active defense challenge more tractable.
Hypersonic weapons possess limitations that ballistic and cruise missiles do not. Besides the aforementioned design challenges, it is significantly more difficult to deploy decoys and countermeasures within the harsh hypersonic environment. Without the need to discriminate warheads from other objects in the cold vacuum of space, hypersonic defense dispenses with one of the most vexing challenges of ballistic missile defense. Hypersonic weapons, being encumbered by aerodynamic drag, may possess longer flight times than ballistic missiles traversing the same distance. Finally, while hypersonic, subsonic, and supersonic cruise missiles can maneuver, hypersonic weapons may present brighter infrared signatures, fly at higher, more detectable altitudes, and may not maneuver as frequently or sharply without losses in performance.
“The characteristic challenges of hypersonic flight raise intriguing possibilities for a defender.”
The characteristic challenges of hypersonic flight raise intriguing possibilities for a defender. By definition, hypersonic gliders expend energy while performing maneuvers. The cost of those actions can be exploited by a defense design that encourages maneuvers early and often (Figure 10). Moreover, the
severe conditions of hypersonic flight—the risk of boundary layer transition, the need for shock wave management—create vulnerabilities that different kill mechanisms can exploit. Hypersonic weapons may be disrupted by smaller impacts or perturbations to their structure or surrounding airflow.
Figure 10: The Cost of Maneuver
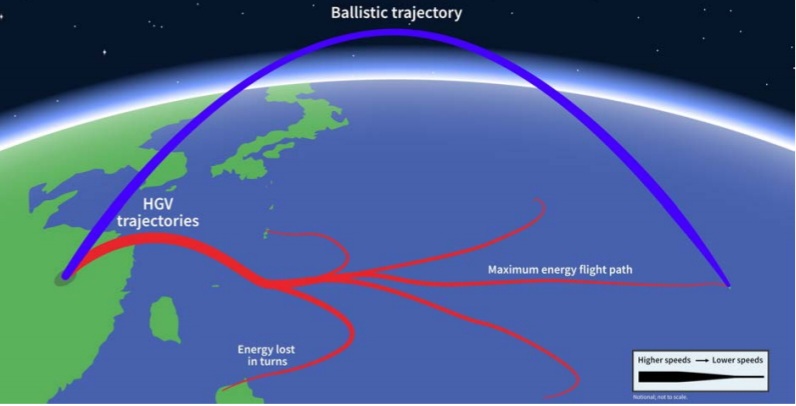
Source: CSIS Missile Defense Project.
Each of the features that give hypersonic weapons an advantage comes with a cost. Extended flight through the atmosphere may expose them to new failure modes. Their ability to maneuver comes at the cost of expending energy and range. Just as ballistic missile defense was oriented around the predictability of a ballistic trajectory, so too can hypersonic defense be tailored to the vulnerabilities of the hypersonic flight regime. “Hit-to-kill” may come to mean something different for defeating hypersonic airframes than it does for ballistic reentry vehicles (Figure 11).
The difficulty of designing and manufacturing hypersonic systems presents yet another vulnerability. Defense architectures that employ multiple defeat mechanisms—kinetic effectors, electronic warfare, and directed-energy systems of various classes and types—would create overlapping headaches for hypersonic weapon designers, who must optimize their designs against a greater variety of effects. Weapons designed with tight performance margins are vulnerable to marginal changes in a defense system’s
characteristics. The need to overcome such uncertainties might force adversaries to embrace more conservative, less capable weapon designs. These dynamics in turn affect the cost of developing hypersonic weapons and the offense-defense relationship more
broadly. A hypersonic defense design that
exploits these “tight tolerances . . . [and]
unique sensitivities that hypersonic weapons must overcome” would impose costs on adversaries.28 In other words, the development and fielding of hypersonic defenses could stress an adversary’s design cadence.
The experiences and program investments
from legacy air and missile defenses can
be leveraged here. These include terrestrial
radar tracking, space-based sensing and
communication, low-latency networking, and battle management modernization. Hypersonic defense can and should emerge out of an evolution of existing frameworks rather than as a new, standalone stovepipe. Given its global
reach and integrated development, today’s
Ballistic Missile Defense System (BMDS) is
the most promising major defense acquisition program to adapt to the hypersonic defense challenge. Uniquely focused on missile defense-centric material development, it also made good sense for Congress to designate the
Missile Defense Agency as the executive agent for hypersonic defense.
Figure 11: Particle Impact Damage
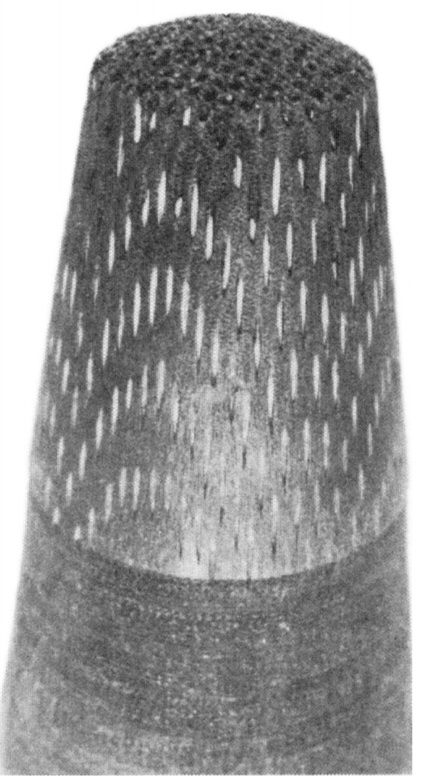
Damage to carbon-composite reentry vehicle nosetip after “flying through rain clouds at 3200 meters/second,” around Mach 10. Source: Sandia National Laboratories. Reprinted with permission.
To be clear, however, converting the BMDS into the Missile Defense System (MDS) will require considerable architectural and cultural change. The “scale and urgency of change required” should not be underestimated. The same degree of reliance upon persistent and dedicated assets may not be available for hypersonic defense as it has for rogue state ballistic missile defense.29 Lessons from the
field of air defense also have purchase here.30 The use of distributed and elevated sensor platforms and forward pickets might be applied to detect lower-flying threats, from hypersonic to subsonic. Adifferent approach to persistence and the employment of non-dedicated elements across the joint
force may also be required.
“Each of the features that give hypersonic weapons an advantage also comes with a cost.”
Hypersonic defense will not be easy, but neither is it impossible. Hypersonic missiles are not silver bullets, and they are not unstoppable.31 By adopting a system-of-systems approach, fielding space sensors and improved interceptors, and employing other imaginative ways to target the unique characteristics of hypersonic flight, the problem of hypersonic defense will be recognizable as a complex but increasingly tractable form of air defense.
The Current Programmatic Context
▪ Hypersonic defense investments have thus far been modest, a small fraction of that devoted to hypersonic strike.
▪ The single most important program element for hypersonic defense is a robust, resilient, and persistent space sensor layer capable of acquiring, observing, classifying, and tracking hypersonic threats.
▪ The second most important program element is a glide-phase interceptor. At the current pace, an effective glide-phase interceptor may not be fielded until the 2030s, leaving vulnerabilities that
adversaries may seek to exploit.
▪ The Missile Defense Agency (MDA) and the U.S. Navy are continuing to develop the Sea-Based Terminal hypersonic defense program as part of the Aegis Combat System. Besides the Aegiscentric
elements, others will also contribute to the mission. The U.S. Army continues to field and will further evolve its air defense portfolio with the Patriot family of interceptors and sensors and battle command system. The Terminal High Altitude Area Defense (THAAD) system has defeated threats in both the high endo- and low exoatmosphere.
▪ Other critical efforts include Next-Generation OPIR, the Space Development Agency Tracking and
Transport Layers, and new command and control investments.
Hypersonic weapons are designed to exploit the limitations of traditional surface-based missile defense tracking and engagement systems. Their defining characteristics—sustained high-speed flight and maneuverability within or at the outer reaches of the atmosphere—threaten to circumvent today’s defense designs. Hypersonic missiles compress the spatial and temporal elements of combat within
which active defenses must perform. These features require a new approach to defense design.
Efforts to develop a hypersonic defense were, until recently, limited to the research and maturation of future concepts. Although Congress designated MDA as the executive agent for developing and
deploying hypersonic defenses in 2016, a funding program line did not appear until fiscal year (FY) 2018.32 Despite synergy between the two efforts, spending on hypersonic defense has been a small
fraction of that for offensive programs (Figure 12). Funding for the program has remained relatively modest over the past three years, primarily focused on architecture studies, early research, and
concept development. In the past year, however, initial design contracts have been awarded for both space sensor development and a glide-phase interceptor.
Figure 12: Hypersonic Defense Budgets, 2018–2025
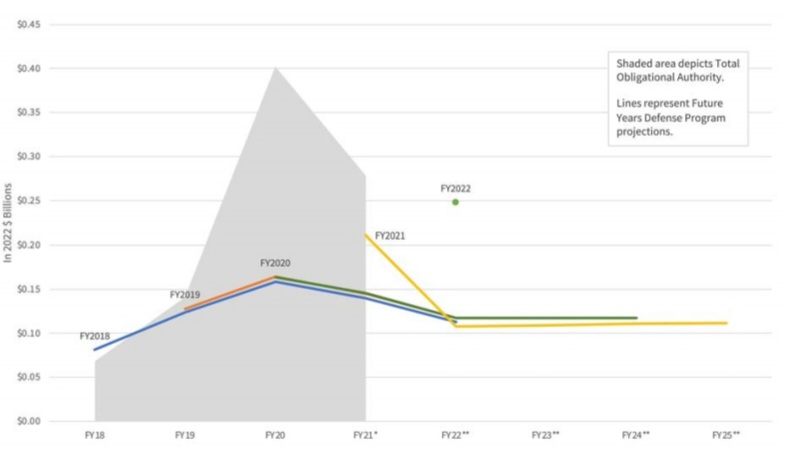
*Appropriated dollars
** Based on previous FYDPs
Source: DoD Comptroller and CSIS analysis.
Under its current roadmap, MDA will develop a limited terminal defense for its near-term effort while also advancing a longer-ranged defense to detect and defeat hypersonic weapons in their
glide phase of flight (Figure 13). MDA is working to evolve existing surface-based radars to initially support hypersonic engagements while also prototyping a sensor payload for a space-based tracking
constellation. Current projects include the Hypersonic and Ballistic Tracking Space Sensor (HBTSS), a Glide Phase Interceptor (GPI), and several technology development efforts. In the longer term, other efforts may also be necessary, including the deployment of interceptors in different domains, faster or longer-range interceptors, and the use of alternative kill mechanisms.
Figure 13: Depiction of Aegis Layered Hypersonic Defense
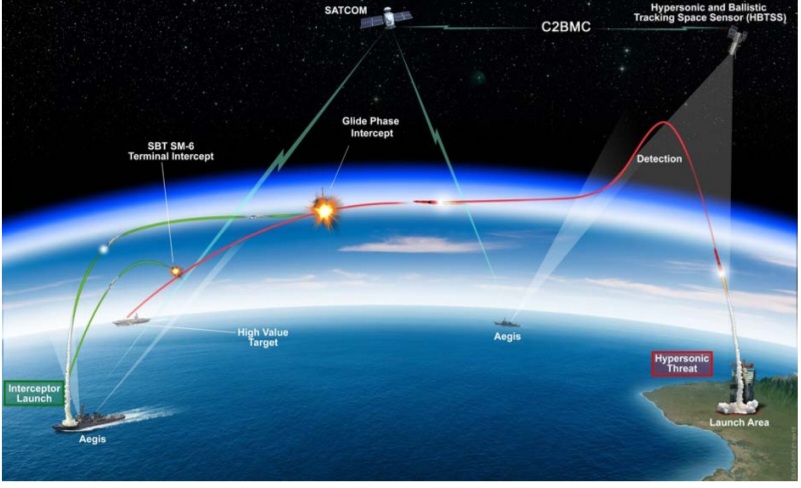
Source: Missile Defense Agency
Space Sensors
Sensing remains the central bottleneck to realizing a hypersonic area defense capability. The current BMDS is dependent on a relatively small number of surface-based radars to track incoming weapons.
Constrained by the horizon, current BMDS sensors can only support counter-hypersonic engagements
in the final phases of flight. The speed of hypersonic weapons leaves little time for computing a fire control solution, communicating with command authorities, and completing an engagement.
Supported by a low-latency communications network, elevated sensors of various kinds are necessary
to resolve the range and mobility challenges associated with surface-based systems. Space-based sensors would enable a “birth-to-death” tracking capability: the ability to follow a hypersonic weapon through the entirety of its trajectory (Figure 14).33 Such a capability would be critical for disrupting or defeating hypersonic weapons early in flight where interception is easier and follow-up shots are possible. A space-based sensor constellation would also aid in targeting adversary missile forces after launch and engaging traditional ballistic missile threats.34 These characteristics make space-based sensors essential for realizing a scalable and comprehensive missile defense architecture.
Today’s strategic space-based sensors for launch detection can provide the BMDS with, at most, limited real-time tracking capability for hypersonic weapons. Legacy missile warning systems in geosynchronous orbit such as the Defense Support Program (DSP) or SBIRS can detect the hot plume of a hypersonic weapon’s rocket booster but may lack the sensitivity to track weapons through their glide phase of flight.35 Although some commentators suggest that existing sensors can detect hypersonic weapons, it is unclear whether they can track their signatures with the resolution and
latency needed to support a firing solution.36 While hypersonic weapons may possess comparatively hot thermal signatures and fly above infrared-attenuating cloud layers, challenges in processing, integrating, and
discrimination may make it difficult for
existing systems to track their trajectories
(Figure 15).37 Fielding a dedicated tracking sensor constellation would fill this gap.
MDA and the Space Development Agency
(SDA) are presently developing several
space-based sensors for the hypersonic
tracking mission. In an architecture named
the Tracking Layer, SDA would produce a
constellation of wide-field-of-view sensor
satellites for launch indication and warning. MDA, meanwhile, continues to develop the Hypersonic and Ballistic Tracking Space Sensor (HBTSS), a satellite constellation capable of tracking hypersonic weapons with the fidelity and latency performance to support intercepts. Based in low earth orbit (LEO), the Tracking Layer’s WFOV satellites could detect hypersonic weapons in their brightest, earliest phases of flight, passing tracking data to HBTSS as they grow dimmer in their glide phase. In October 2020, SDA awarded $342 million to two contractors to construct the first eight WFOV satellites.38 These satellites, designated Tranche 0, are
scheduled for launch by 2022 to 2023. The next sets, designated Tranche 1 and further, are expected to enter service between 2024 and 2026.
Figure 14: Space-Based Sensors Enable
Low-Altitude Target Tracking
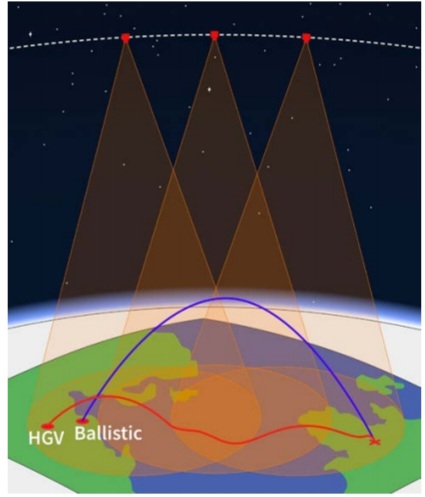
Source: CSIS Missile Defense Project.
With its higher sensitivity and medium field of view, HBTSS would provide the detailed, low-latency data—“fire-control-quality data”—needed to support glide-phase intercepts.39 Prototype HBTSS sensors could be deployed on dedicated platforms, while operational units would be hosted on SDA developed platforms or commercial satellites.40 Previous defense budget requests attempted to transfer
responsibility for HBTSS to SDA, but successive National Defense Authorization Acts (NDAAs) have maintained the program under MDA direction, as did the 2022 Presidential Budget request.41
Figure 15: Sensing Challenges in Ballistic and Hypersonic Missile Defense
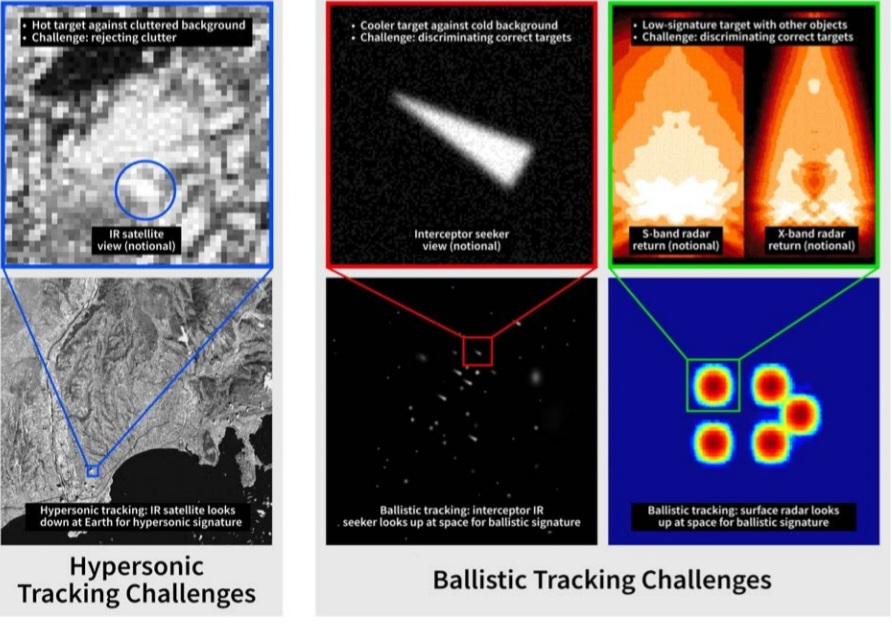
Left: Notional depiction of challenges associated with space-based hypersonic tracking. Center: Challenges associated with endgame tracking during ballistic intercept. Right: Notional depiction of challenges associated with ballistic midcourse tracking.
Graphic: CSIS Missile Defense Project. Photo credits: European Space Agency (top and bottom left); Missile Defense Agency (top and bottom right); Masao Dahlgren, CSIS (top and bottom center).
The FY 2021 NDAA requires MDA to begin orbital testing of the HBTSS sensor by December 2023, after which it “shall achieve full operational deployment of such sensor payload” as part of SDA’s Tracking Layer.42 In total, lawmakers transferred SDA’s requested $20 million for HBTSS to MDA and awarded an additional $100 million to the overall program of record for FY 2021.43 SDA plans to operationalize a regional hypersonic tracking capability with approximately 150 satellites by FY 2025.44 To that end,
SDA requested $287 million and $260 million toward LEO sensing and communications satellites, respectively, for FY 2022 (Figure 16).45
A space-based hypersonic sensor layer could leverage advances in infrared sensors, image processing, and machine learning to make birth-to-death tracking feasible. In an analysis of alternatives, MDA evaluated several satellite constellation types sized for low-, medium-, or geosynchronous orbits
(LEO, MEO, and GEO, respectively) for this purpose. Although fewer satellites would be needed to provide regional coverage in GEO orbits, their distance from Earth would incur higher launch costs and require larger, more sophisticated sensors and communications payloads. A larger constellation of LEO satellites, by contrast, could leverage recent advancements in commercial LEO access to dramatically
reduce unit cost, although such orbits require comparatively more satellites.46 Orbiting closer to threat trajectories, such satellites could use lower-resolution sensors than GEO systems to achieve
comparable resolution. In addition, their larger numbers—at minimum around 50 small satellites to stereoscopically track hypersonic weapons—could offer more resilience to attack than a smaller
number of satellites in higher orbits.
Figure 16: Selected Defense-Wide Space Sensor Programs, 2003–2022
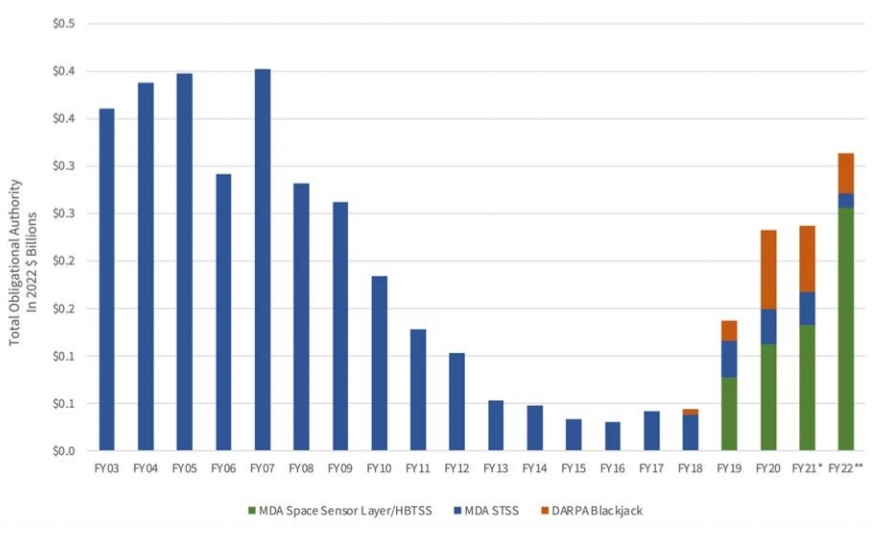
*Appropriated dollars
** Based on 2022 PB
Source: DoD Comptroller and CSIS analysis.
Despite promising initial steps, barriers remain to realizing a LEO hypersonic tracking capability. Although a large constellation of dedicated LEO satellites and commercially hosted sensors would pose a challenging target set, deploying sensors in multiple orbits, including in MEO, might exacerbate an adversary’s targeting dilemma. Proliferation is not the only way to achieve resilience; diversity across orbits helps as well.
Ground control and rapid data transmission between so many elements could pose a secondary challenge to realizing a LEO-based or mixed-orbit sensor architecture; smaller satellites possess less power generation capability for the high-bandwidth, low-latency communications necessary for missile tracking. Integrating sensor data from a large constellation of smaller satellites will require enhanced inter-satellite communications and processing capability. Fixed ground terminals, moreover, could be vulnerable to disruption or attack. The success of such efforts will depend heavily on recent commercial innovations in LEO satellite communications, signal processing, and edge computing, which could reduce the latency and size of data transmissions and the necessity of separate, vulnerable
data processing centers on the ground.47
Interceptor Development
Today’s active missile defenses largely depend on space-based infrared detection and surface-based radar tracking as a ballistic missile rises over the curvature of the Earth and into space. Barring minor
course corrections or atmospheric friction, ballistic objects will follow a trajectory dictated by gravity. Missile defenses generate fire control solutions and task interceptors to engage the target at some
desired point along that same route. Ballistic missiles’ relative predictability allows ballistic missile defenses to operate with a larger margin of latency and tracking error than hypersonic weapons. Current interceptors such as the Standard Missile-3 (SM-3) use this advance time to intercept a ballistic missile at extended distances downrange, in their exoatmospheric midcourse phase.
Flying closer to the Earth, hypersonic weapons remain beneath the tracking horizons of today’s surface-based radars (Figure 17).48 Even if hypersonic weapons could be tracked during their cruise
or glide phase of flight, current midcourse interceptors—designed to operate in the exoatmospheric environment rather than the harsher endoatmospheric regime—would not be able to engage them.49
With the current architecture, the first opportunity to develop a fire control solution for hypersonic missiles is in their final phase of flight, the terminal phase, when they are especially elusive. Today’s
air and missile defense interceptors, designed for slower or more predictable targets, lack the kinematic and divert performance to reliably intercept terminal-phase hypersonic maneuverability
(Figure 18). One oft-quoted rule is that an interceptor requires three times the maneuvering acceleration of its target.50 While some interceptors have “demonstrated performance against . . .
advanced maneuvering threats” in the high atmosphere and just above it, kinetic terminal intercept is a stressing challenge.51
Figure 17: Hypersonic Weapons Underfly Surface Sensors
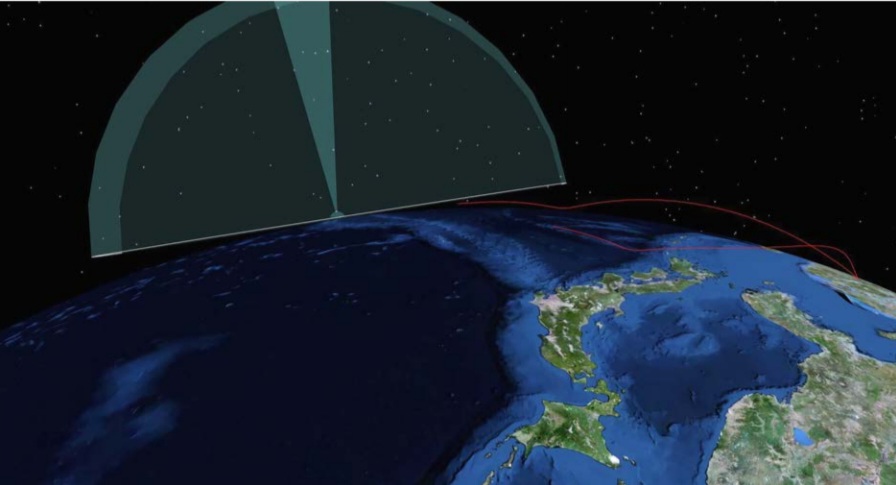
Notional depiction of hypersonic trajectories underflying detection horizon. Source: CSIS Missile Defense Project. Image created using SMARTset, a modeling and simulations tool for air and missile defense analysis
Figure 18: Gaps in Current Layered Architecture
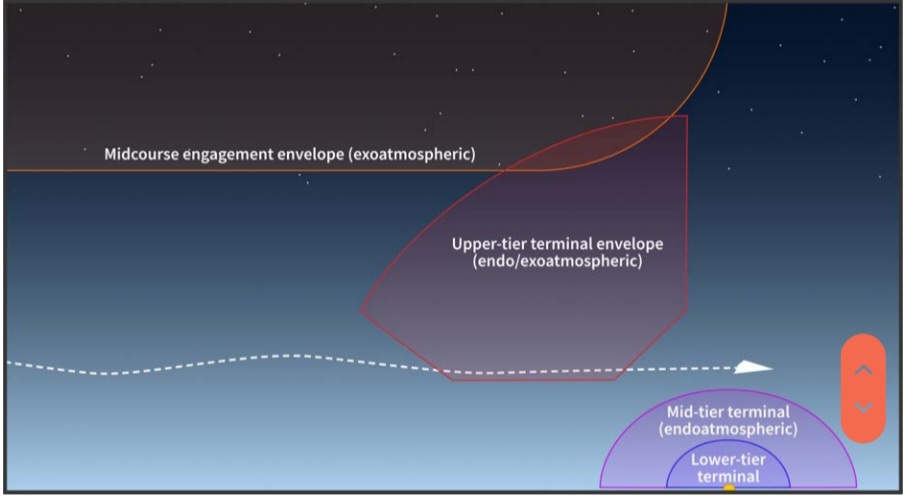
Source: CSIS Missile Defense Project.
For this reason, MDA director Vice Admiral Jon Hill has said that terminal intercept is “never where we want to be.”52 More favorable options are available by engaging hypersonic weapons in the earlier glide phase of flight, where they are less likely to expend energy on sharp evasive maneuvers. Glide-phase interception will be critical for reliably defeating these challenging targets.
Options may yet remain for bolstering terminal defenses against hypersonic weapons. By incorporating new basing modes, mission planning, and sensor and interceptor upgrades—and by imposing costs on the threat in other ways—terminal systems may be adapted to face the hypersonic challenge. For now, however, focus is rightly on glide-phase intercept.
The challenge of intercepting hypersonic weapons in the terminal phase points to the need to detect and engage hypersonic weapons in earlier phases of flight, where they are more vulnerable. Long-range defenses are useful for securing deployed forces across larger areas and for exploiting hypersonic weapons’ kinematic weaknesses in midcourse flight. Hypersonic weapons in their glide
phase maneuver significantly less to conserve energy.53 A long-range interceptor, remotely cued by space sensors to permit earlier launch, offers a larger battlespace, more warning time, and the ability to employ shoot-look-shoot shot doctrines. Besides the primary effect of alleviating the terminal intercept challenge, longer-range intercept capability may have the secondary benefit of encouraging
the threat to employ circuitous routes and earlier maneuvers that diminish endgame performance.
New long-range interceptors will be required to kill hypersonic missiles in their glide phase. Midcourse ballistic missile interceptors such as the SM-3 and Ground Based Interceptor are designed to engage ballistic missiles in the vacuum of space, not in the atmosphere. While existing terminal effectors, such as the
Standard Missile-6 (SM-6) and THAAD, have engaged targets in in the high atmosphere, their performance against hypersonic targets is yet to be fully
characterized (Figure 19). Developing a long-range glide-phase effector will present different technical demands than for midcourse ballistic missile interceptors. The design of infrared
seeker windows that can survive hypersonic environments, for example, has historically posed a challenge for the development of terminal, endoatmospheric interceptors.54
Figure 19: THAAD Transatmospheric
Engagements
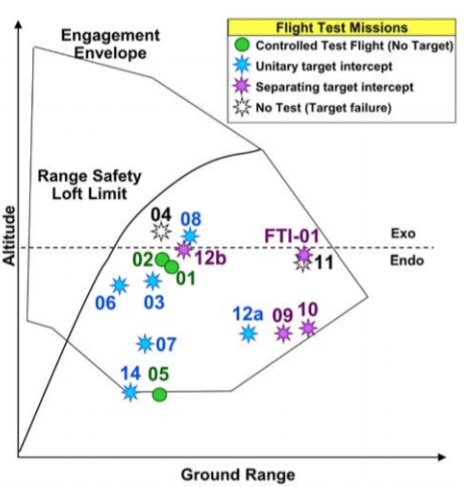
Source: Lockheed Martin Corporation, dated March 2013. Reprinted with permission.
Sea-Based Terminal
MDA currently maintains two interceptor
program efforts, focused on terminal- and
glide-phase intercept, respectively. The SeaBased Terminal (SBT) system, developed to intercept high-speed maneuvering reentry vehicles, is the first U.S. effort at hypersonic defense. Initially deployed in 2018, SBT consists of an Aegis Baseline 9 destroyer and the SM-6 interceptor and is intended to defeat maneuvering and ballistic threats in their terminal phase of flight. The Navy and
MDA first validated the SBT Increment 1 system against a maneuvering target in flight test FTM-27 Event 1 in December 2016.55 MDA successfully concluded a second test, FTM-27 Event 2, in August 2017 after certifying an upgraded SBT Increment 2 capability in September 2018, MDA planned to test the system against a medium-range ballistic missile, aircraft, and unspecified threat in a series of
flights through FY 2019 and FY 2020. These tests, designated FTM-31, were delayed until FY 2021 due to the coronavirus pandemic and other unspecified issues.56
Further evolutions will be necessary to allow SBT to address more advanced hypersonic threats. MDA plans to deliver an additional upgrade to the system, SBT Increment 3, by FY 2024.57 The agency intends to test SBT against a “next-generation hypersonic-threat representative target” in FY 2023.58 In April 2021, Acting Under Secretary of Defense for Research and Engineering Barbara McQuiston testified that MDA would test an “SBT capability to address the regional hypersonic threat” in FY 2024.59
“Hypersonic vehicles themselves are relatively fragile during their long phase of cruise flight. They’re fairly easy to destabilize. They’re in a very difficult flight regime, and there, decoys are not possible. They glow brightly in the infrared. If they’re going to reach their target, they have to be in relatively straight-line trajectories. Yes, they can maneuver, but they can’t maneuver in their cruise phase as easily as an interceptor can maneuver.”60
— Michael Griffin, Former Under Secretary of Defense for Research and Engineering
Glide Phase Interceptor
MDA has also initiated efforts to realize a glide-phase hypersonic defense by the late 2020s.61 In August 2020, Vice Admiral Hill noted that glide-phase flight was “where the hypersonic threat is its most vulnerable . . . where it’s bleeding energy, not maneuvering as much,” and is “easier to track” than in the terminal phase.62 Longer-range defenses would also offer longer warning times and the potential to conserve interceptors by firing them sequentially over time (shoot-look-shoot) instead of simultaneously in the final phases of flight.63 Engaging hypersonic threats earlier in flight will be necessary for area-wide defense rather than point defense.
In its early iterations, this notional architecture would incorporate forward-based Aegis destroyers and early HBTSS satellites to detect hypersonic weapons, and a new glide-phase interceptor to engage them at range. In 2018, MDA commissioned 21 white papers exploring counter-hypersonic weapon concepts, and in January 2020, it issued a request for proposals to integrate what was then called a Regional Glide Phase Weapon System (RGPWS) interceptor into the Aegis Combat System. The request for proposals notably asked for information relating to the “potential for multi-mission capability.”64
After reviewing industry proposals, MDA announced in August 2020 that it would restructure RGPWS to prioritize concepts that could be fielded by “the mid-20s.”65 On May 19, 2021, MDA issued its first
solicitation for a newly titled program, the Glide Phase Interceptor (GPI) Initiative. The shift to GPI reflected the intention, in the words of Michael White, to “parallelize the technology development and weapon system development” and thus accelerate the fielding of capability.66 Whether such concurrent efforts incur developmental risk depends upon the nature and maturity of the several efforts.
Funding requests and appropriations for GPI have grown from $66 million in FY 2020, to $127 million in FY 2021, to $136 million requested for FY 2022 (Figure 20).67 At this development pace, however,
GPI fielding would probably not be expected before the end of the decade. In November 2021, MDA awarded initial competitive contracts for GPI to three separate contractors.68 While GPI will initially be fielded at sea, it could be based on land as well.69
An MDA video of the GPI concept depicts sufficient time for a shoot-look-shoot firing doctrine, but it may be challenging given the limitations of the Navy destroyers’ vertical launcher size and the absence
of direct space-to-weapon datalinks.70 A larger effector and launcher, or alternative launcher platforms, may be required for deeper reach and shoot-look-shoot capability.
Separately from both GPI and RGPWS, the Defense Advanced Research Projects Agency (DARPA) has continued to mature a “critical component technology” for glide-phase intercept. Its Glide Breaker
effort, established in 2018, received $27 million in FY 2019 and aimed to conclude bench testing in FY 2021.71 Glide Breaker efforts could combine with or at least inform future GPI progress.
It remains unclear if GPI will ultimately be a hit-to-kill weapon or use an alternative kill mechanism. A blast-fragmentation warhead might be good enough, for instance, to “throw it off course” or
cause catastrophic failure with lower kinematic requirements.72 Weapons that damage or disrupt hypersonic weapons earlier in flight might lower the challenge facing terminal defenses. In the
harsh environment of sustained hypersonic flight, explosive shockwaves or even small heat shield punctures could destabilize or destroy a vehicle. As blast-fragmentation warheads do not need to directly strike the target to damage it, they may offer “relief in [the] error budget” for guidance and divert requirements, but there are trade-offs in size and weight.73 Regardless of whether their kill mechanisms are blast-fragmentation or hit-to-kill, kinetic interceptors would benefit from additional effects that slow the threat and reduce its freedom of action.
“We’ve had lots of debates internally over ‘is hit-to-kill necessary?’ There’s a lot of
benefits in imparting kinetic energy on an incoming missile; whether it’s conventional or nuclear, there tends to be a massive destruction once you hit it with that force, that imparts that momentum into it. But if you’re trying to just get it off that glide phase, you could probably get away with less than hit-to-kill. So, we are into precision guidance because we always want to assume that’s where we want to go, and we continue to work the precision guidance aspects of glide phase. But there will be some relief in that error budget if we decide to go with some sort of conventional type warhead as opposed
to a hit-to-kill frontend.”74
— Vice Admiral Jon Hill, Director, Missile Defense Agency
To mitigate costs and accelerate development, the United States could also leverage existing assets and production lines for long-range hypersonic effectors.75 Current booster designs would reduce integration risks with launch platforms and exploit economies of scale. Software modifications, seeker enhancements, and modified propulsion for THAAD, PAC-3 MSE (Missile Segment Enhancement), and the Standard Missile family might offer additional capability for shorter-range glide-phase intercept.
A comprehensive approach to hypersonic defense could incorporate these and other systems to channel the threat—to exploit kinematic vulnerabilities to maximum effect. The single most important
program element of such a defense remains the development and fielding of a space-based sensor layer, followed by a glide-phase effector system to mitigate the threat at greater range.
Command and Control
Hypersonic defense poses significant geographic and domain challenges that could strain command, control, and communication. The problem is not unique and is related to those encountered with
ballistic missile defense and other forms of air defense. Rather than developing a new, standalone stovepipe, MDA is pursuing adaptations to the existing Command, Control, Battle Management, and Communications (C2BMC) system. MDA has so far invested in leveraging and upgrading the C2BMC system to create a contingency capability for hypersonic detection and warning. C2BMC Spiral 8.2-5, planned for FY 2022, will include an initial situational awareness and tracking capability for hypersonic threats along with its integration of the new Long Range Discrimination Radar. Future upgrades and
evolutions for hypersonic defense will require additional upgrades to C2BMC.
Vast troves of data could be leveraged to develop better decision aids. Presently, various combatant commands cannot process the substantial majority of collected radar, flight test, or shared intelligence data—challenges that motivated the Department of Defense’s Joint All Domain Command and Control
(JADC2) program.76 In recent demonstrations, U.S. Northern Command has developed new software tools to process multiple intelligence streams and detect missile launch preparation activities several days in advance.77 Data collected from the United States’ own hypersonic flight tests and modeling efforts could further inform the development of enhanced analysis tools. Recent initiatives point in the direction of software that can fuse geospatial and weather information, synthetic hypersonic flight models, and other data streams to generate solutions for sensor and effector placement, deployment tempo, shot doctrine, and other aspects of the hypersonic defense mission. C2BMC has already
demonstrated forms of joint, all-domain command and control and thus will play an important role in the development of future JADC2 efforts.
Budget Outlook
Limited budgets for hypersonic defense have been symptomatic of an ongoing squeeze on missile defense research and development, but this may be changing.78 In its 2020 Unfunded Priority List, MDA included an additional $720 million in supplemental funding, suggesting that accelerated development was crowded out by other programs.79 MDA’s Unfunded Priority List for FY 2021 included an additional $224 million for hypersonic defense activities and $62 million for FY 2022. Each year, Congress provided a portion of MDA’s unfunded priorities but did not award the full amounts (Figure
20). At this level of investment and development, glide-phase intercept may not appear until the end of the decade, or even the early 2030s. Should funding for hypersonic defense increase in FY 2023 and FY 2024, an operational hypersonic defensive capability could be available by around 2028.
Figure 20: Hypersonic Defense Unfunded Priorities and Appropriations
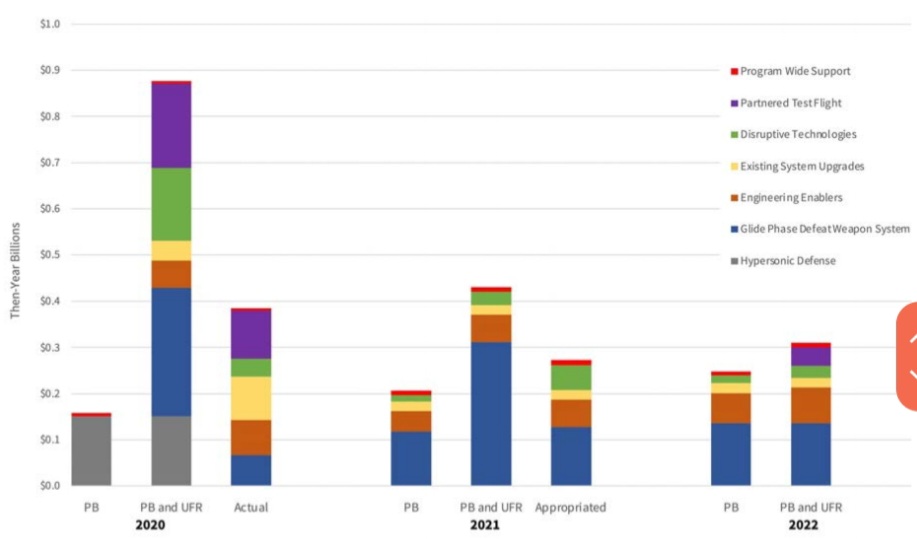
Source: CSIS Missile Defense Project.
Channeling the Threat
▪ Effective hypersonic defense will attack the unique characteristics of hypersonic flight. Because hypersonic weapons expend energy by conducting maneuvers, the defensive job could be made easier by encouraging earlier or more frequent maneuvers and by exacerbating the thermal and aerodynamic stresses of hypersonic flight.
▪ Alternative and forward-based deployments of surface-based radars and alternative kill mechanisms could impose costs on the threat and encourage early maneuvers.
▪ Alternative, distributed, and redundant basing modes could reduce adversaries’ confidence in their ability to construct an effective attack.
▪ The single most important program element for hypersonic defense is a space sensor layer. Other elevated and distributed sensors can contribute to increasing warning and cueing time, especially before a full space sensor layer is fielded.
Hypersonic weapons are fast but do not quite turn on a dime. If high-speed maneuverability is a key feature of hypersonic missiles, it is also a potential weakness. Intense heat and drag impose
limits on their ability to maneuver at high speeds. Unpowered hypersonic gliders necessarily expend energy in maneuvers, compromising speed and range with each successive turn. While hypersonic cruise missiles can expend additional fuel to recover speed after turning, their maneuver envelope is constrained by the aerodynamics of scramjet engines, which are sensitive to airflow disruptions
induced by sharp turns. Every maneuver comes with a cost.
The fact that hypersonic gliders try to conserve energy for later maneuvers is what makes glide phase intercept attractive. As Vice Admiral Jon Hill has emphasized, “The hypersonic threat is
not invincible—in that [glide] phase, it’s bleeding off energy.”80 After space sensors, a glide-phase interceptor remains the highest programmatic priority. A comprehensive defense design could
go further, using sensors, effectors, and employment concepts to encourage the threat missile to hemorrhage energy.
Such an approach would channel the threat. Forcing adversaries to plan more circuitous routes or evasive maneuvers could thereby increase warning time, create uncertainty, diminish threat weapon
performance, and, in turn, reduce the burden on the inner layers of a hypersonic defense design.
Encouraging Maneuver
Existing defensive assets could be placed in locations that force adversaries to plan maneuvers. While today’s architecture of surface-based radars and terminal interceptors cannot detect and engage
hypersonic weapons at extended ranges, a defender might extract additional performance from the Sea-Based Terminal system or others by diversifying and obscuring their basing locations. Other sensors and interceptors could be based in domains other than at sea and in locations that stress an adversary’s mission planning.
Although hypersonic missiles do not have nearly so predictable a trajectory as ballistic missiles, there are, nevertheless, more and less optimal routes for energy and time conservation. Modeling these
routes can in turn support the construction of a defense plan and the siting of sensors, interceptors, and alternative kill mechanisms.
On the sensor side, a complete picket fence of radars in the Pacific is not necessary to have such an effect. A distributed and redundant sensor defense architecture that includes advanced digital radars could emphasize surface radar placements that force attackers to either bleed energy or accept earlier detection. Either choice could increase early warning for an attack and make engagements more effective. Over-the-horizon radars may provide earlier cueing as well.
Enhanced modeling and simulation will be needed to optimize such defenses. Advanced threat modeling software incorporated into C2BMC could aid by anticipating potential adversary launch
positions and trajectories, in turn optimizing the placement of defense elements. Artificial intelligence and machine learning tools can optimize sensor and weapon resource utilization for
future defense architectures.81
Figure 21: Forward-Based Elements Affect Maneuver Budgets
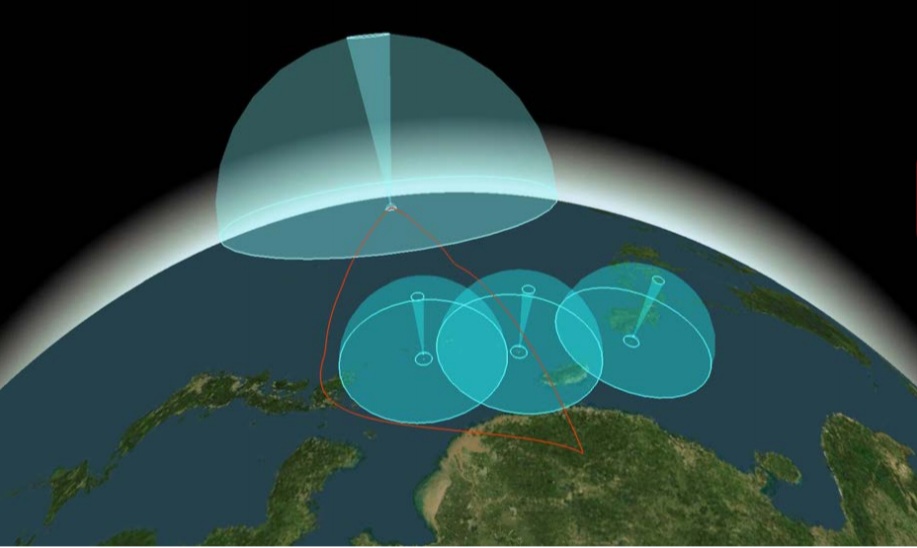
Notional depiction of hypersonic glide trajectories with impact of avoiding forward sensors. The direct path has a flight time of 928 seconds. The more circuitous route has a flight time of 1,360 seconds.
Source: CSIS Missile Defense Project. Image created with SMARTset, a modeling and simulation tool for air and missile defense analysis.
The hypersonic missile defense challenge is a complex air defense challenge for which historical lessons may be instructive. The problem of low altitude aerial attack has spurred imaginative ideas
to thwart it. During World Wars I and II, Great Britain deployed extensive “balloon aprons” around London (Figure 22). The balloons held aloft extensive steel nets to impede the flight of low-flying aircraft and, later, to drive German bombers to higher altitudes. During World War II, over 2,000
balloons were deployed around London and maintained until the end of the war, with a separate Balloon Command created for their maintenance. Some 231 V-1 missiles were reportedly destroyed by
these balloon aprons, as were numerous German aircraft.82
Barrage balloons were by no means a perfect defense, and by definition they could be circumvented by going higher in altitude. But they had an effect on German mission planning and forcing manned
bombers to go higher made them less accurate and more vulnerable to antiaircraft flak. A similar logic applies to complicating hypersonic strike. To be sure, balloon screens as such would not be useful against advanced missile threats today. The point here is to highlight an analogy for channeling the hypersonic threat.
Figure 22: Balloon Apron around London, 1915
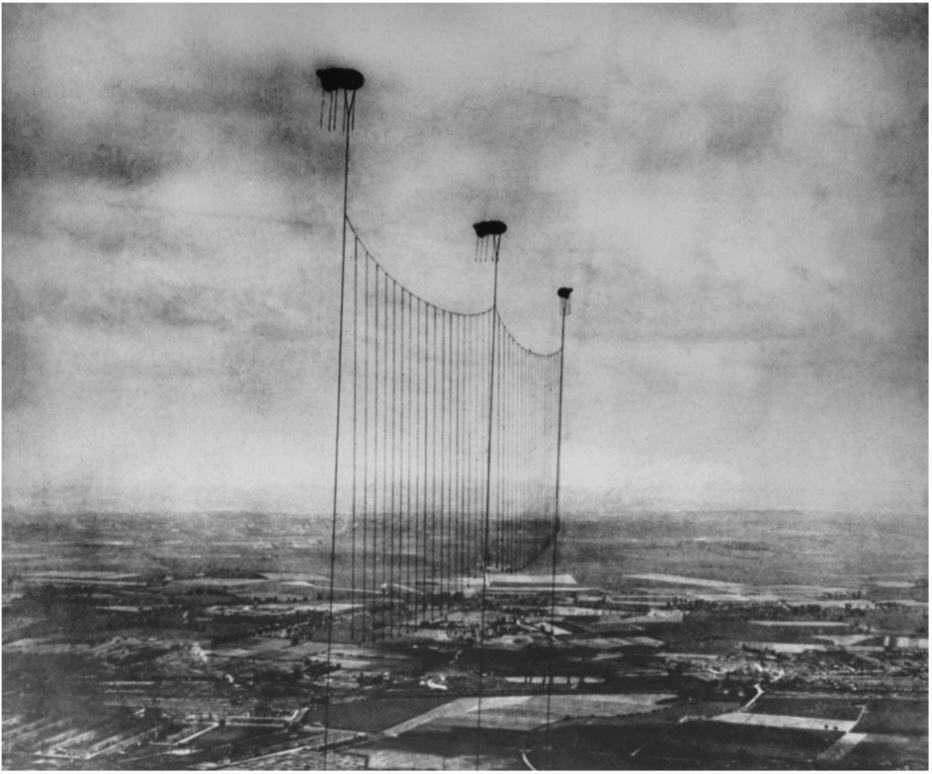
Photo by © Hulton-Deutsch Collection/CORBIS/Corbis via Getty Images.
Mobility and Distribution
As with the air defense mission, a hypersonic defense design would benefit from making greater use of mobile and more distributed elements. By using mobile systems, the Joint Force could more rapidly rotate assets to optimal positions and reduce adversaries’ ability to preemptively target them. More importantly, mobile elements would allow the Joint Force to rotate defensive assets closer to prospective launch sites, limiting the set of trajectories where an adversary’s weapon could maneuver to avoid engagement.83
Air-based sensors and weapons offer improved mobility, leveraging mature technologies and offering greater range, survivability, and flexibility. With their faster transit times, aircraft could be rapidly retasked for point-defense applications. Moreover, off-the-shelf airframes, effectors, and radars already exist for
engaging airborne targets, and industry retains a considerable institutional knowledge in integrating airborne sensor platforms. The Navy has already integrated airborne sensors, for example, into its Aegis data ecosystem, a capability named Navy Integrated Fire Control-Counter Air (NIFC-CA).84 The Navy also reportedly demonstrated an FA/18F-integrated SM-6 missile in 2018.85 In 2021, the Army demonstrated a similar capability, passing tracking data from an F-35 via its Integrated Air and Missile Defense Battle
Command System (IBCS) to a Patriot missile battery, successfully intercepting a cruise missile target.86
Loitering airborne platforms carrying interceptors, sensors, or alternative kill mechanisms could also increase the range of a defensive system. Kinetic interceptors benefit from being launched at higher
altitudes, conserving the disproportionate amount of fuel needed to accelerate from the surface and through the thicker lower atmosphere. Most surface-based sensors remain limited by the Earth’s curvature in detecting and tracking hypersonic weapons, which become visible within several hundred kilometers and leave only minutes of time to react. An airborne platform could extend the radar line
of sight by multiples and could incorporate infrared or other non-radar sensors that cannot function effectively at lower altitudes.87 Multiple aircraft or unmanned platforms would be needed to maintain
continuous coverage.88 Orbits located further from airfields would increase the number of aircraft required, as more flight time would be spent transiting to the patrol area. With higher on-station endurance and lower maintenance and cost, high-altitude unmanned aerial vehicles (UAVs) would
likely be preferable to tactical aircraft. Prior assessments have asserted that UAV-borne airborne patrols using mature infrared sensor technology might cost up to $5 billion for three defended areas
over a 20-year life cycle.89
Distributed or concealed platforms would add further dilemmas for adversary mission planners. In the event of heightened indications and warning, containerized sensors, interceptors, and alternative kill mechanisms could be placed at numerous locations alongside decoys, as part of a giant shell game.90
Such deployments would not be persistent, but the capability to distribute them in or during a crisis could complicate adversary planning. Improved software tools would allow senior decisionmakers to select enhanced deployment patterns. Mobile basing platforms—aircraft, surface vehicles, and ships—could rapidly rotate capability and sap adversaries of initiative, forcing them to react.
To be sure, these are not defense design features commonly associated with the legacy BMDS. Such deployments would probably not be persistent and would not be dedicated assets in the way that
major BMDS elements are for rogue state ballistic missile threats today. It presupposes the presence of, and integration between, joint and combined capabilities in areas such as the Indo-Pacific that would not be specifically tasked to the hypersonic defense mission. While MDA has a unique role for
hypersonic defense-specific elements, other organizations and forces must contribute as well. Such a comprehensive approach becomes possible if the hypersonic defense mission becomes a joint priority.
Exploiting New Failure Modes
▪ The stresses of the hypersonic flight regime make it possible to disrupt or disable hypersonic missiles with a variety of weapon types and by exploiting a variety of failure modes.
▪ Besides hit-to-kill, area-wide kill mechanisms might include high-powered microwaves and particle-dispensing warheads as a kind of twenty-first century flak. The utility of laser weapons is
less certain.
▪ Multiple kill mechanism payloads, delivered via modular booster rockets or other platforms, could encourage adversaries to redesign hypersonic strike weapons, perhaps at significant expense or design cost.
Technical challenges associated with developing effectors for area-wide hypersonic defense should compel the United States to evaluate alternative kill mechanisms. The violence of the hypersonic flight regime presents opportunities for alternative approaches quite unlike today’s Ballistic Missile Defense System (BMDS). A hypersonic weapon’s operating envelope is more susceptible to disruption than other missile systems. This may make it possible to achieve lethality with several types of effects.91It may also encourage different area-wide effects and thus a new application of “detect-controlengage.”92
In the hypersonic flight regime, impacts from even small particles can damage or destabilize an airframe. Absent heavy mitigation measures, disruption of the hypersonic flowfield could lead to
progressive deterioration of performance or a mission kill, if not outrightcatastrophic failure.93
Electrical, optical, thermal, or electromagnetic disruptions from directed-energy systems might stress the tight design margins of hypersonic weapons.94 Although adversaries will design hypersonic weapons to survive varied and harsh environmental conditions, novel particle and directed-energy
technologies could compel adversaries to employ more conservatively-designed, heavier, or lowerperformance
hypersonic systems. Such approaches could supplement new terminal and glide-phase effectors, supporting a diverse and layered defense with the affordability and scale needed to defeat larger numbers of threats.
Area-Wide Effects
Hit-to-kill intercept has become the preferred kill mechanism in modern ballistic missile defense. Compared to nuclear-armed interceptors or interceptors with conventional blast-fragmentation
warheads, hit-to-kill warheads promise catastrophic destruction of the target, making it easier to confirm whether a successful interception took place. The ability to rapidly verify successful
interceptions is critical to shoot-look-shoot firing doctrines, which conserve interceptors by firing second shots only when initial misses are verified.
In days gone by, defenders offset the inability to precisely track and intercept ballistic missile reentry vehicles by arming interceptors with nuclear weapons as the kill vehicle. The nuclear payload of these interceptors—essentially, massive undirected energy weapons—would affect a wide area near where reentry vehicles might be expected. Both the United States (briefly) and the Soviet Union deployed nuclear-armed interceptors; Russia still fields them today outside Moscow. Similar means were employed for air defense. Nuclear-armed air-to-air missiles were devised and fielded on the principle that, with early warning, interceptor aircraft could be scrambled and might get close enough to effectively destroy an incoming bomber fleet. Since the Strategic Defense Initiative, missile defense efforts have developed more advanced tracking and precision guidance methods. Although mass energy payloads remain in the realm of possibility, nonnuclear missile defense efforts have become a kind of mainstay. Some early hit-to-kill interceptors had expanding blades or nets—kill enhancement devices—to compensate for miniscule course errors in the endgame.
If verifying a direct hit on ballistic threats was challenging, doing so for a maneuvering hypersonic weapon is more difficult. This challenge could be met in part with more capable and agile interceptors or by interceptors that can reach out earlier into glide phase. There may also be opportunities to approach it with alternative effects.
Without recourse to a nuclear blast or other mass energy device, other area-wide effects could be used to compensate for target uncertainty. Given the significant agility required to cover a hypersonic
weapon’s possible maneuver envelope, it may become practical to employ warheads with area effects that could compensate for these weapons’ positional uncertainty. A hypersonic weapon might be unable to complete its mission even if it is not catastrophically destroyed. Different kill mechanisms could exploit hypersonic weapons’ sensitivity to aerodynamic disruptions and particle impacts. A
future glide-phase effector could even employ a modular approach, able to alternately load various payloads to defeat hypersonic threats.
Figure 23: Area-Wide Effects to Relieve the Error Budget
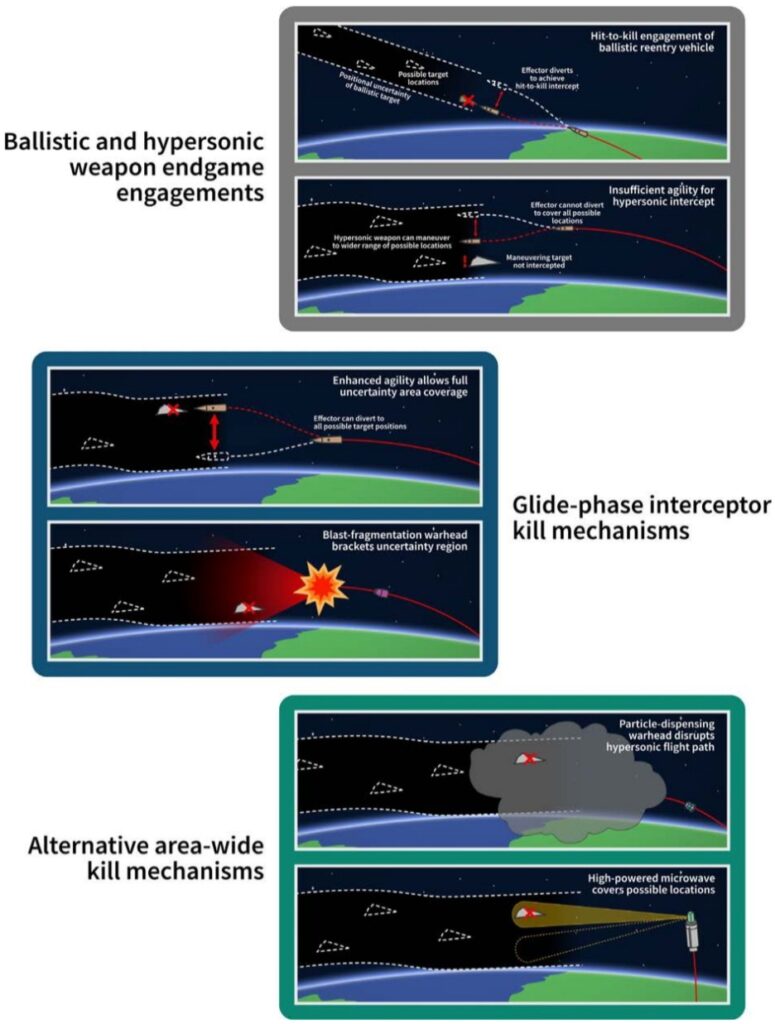
Source: CSIS Missile Defense Project.
Twenty-First Century Flak
Defense against highly maneuvering hypersonic missiles may require area-wide effects. Here, “layered defense” takes a form different from its legacy conception of a linear sequence of shots, one after
another. Different layers or kill mechanisms do not merely catch what a previous layer missed, but cumulatively stack together to degrade a given threat. Instead of relying only on a fast, single-purpose interceptor with an extremely agile kill vehicle, interceptors with alternative payloads may be able to
together present hypersonic weapons with multiple challenges.
One such possibility is a twenty-first century version of “dust defense.” Missiles or airborne platforms could dispense particulate matter to disrupt or destroy hypersonic weapons. At hypersonic velocities, missile impacts against atmospheric dust, rain, and other particles can deposit bullet-like kinetic energies, triggering unpredictable aerodynamic, thermal, and structural disruptions.95
Past efforts to develop ballistic missile reentry vehicles expended significant resources on mitigating atmospheric and weather-related erosion. Studies from decades ago found that as ballistic warheads reentered the atmosphere, atmospheric particles, rain, dust, and other debris “removed significant nosetip material.”96 These weather erosion phenomena reportedly caused significant degradations in accuracy, driving research on enhanced reentry vehicle materials and employment concepts.97 The United States had previously sought to exploit this phenomenon to protect its land-based ICBMs. In a concept named Dense Pack, planners hypothesized that a field of ICBM silos could be safeguarded via “dust defense,” where nuclear explosions from enemy fratricide would form clouds of soil dense enough to degrade or destroy subsequent reentry vehicles.98
The periods of time hypersonic weapons spend in the atmosphere could increase their vulnerability to particulate-based environments. A hypersonic “dust defense” would not generate tons of heavier
debris in the final seconds of reentry. Instead, the defender might dispense engineered particles across a broader airspace early in a hypersonic missile’s trajectory, allowing damage to compound over time. The principle would be to temporarily saturate the domain in which hypersonic flight can take place.
Engineered with a sufficiently wide dispersal pattern, such an effector could operate with simpler guidance and agility requirements than blast-fragmentation weapons. Metallic, pyrotechnic, or other
purpose-designed particles could dwell for tens of minutes in the upper atmosphere, relaxing timing requirements for hypersonic engagements. Such effects would be temporary, but much of the concern about hypersonic missile attack relates to a short, highly concentrated, and structured attack. Given the higher speeds earlier in the flight of a hypersonic glider, a “wall of dust” would be more effective
earlier rather than later in its flight.
Figure 24: Weather and Particle Impact Energies by Speed
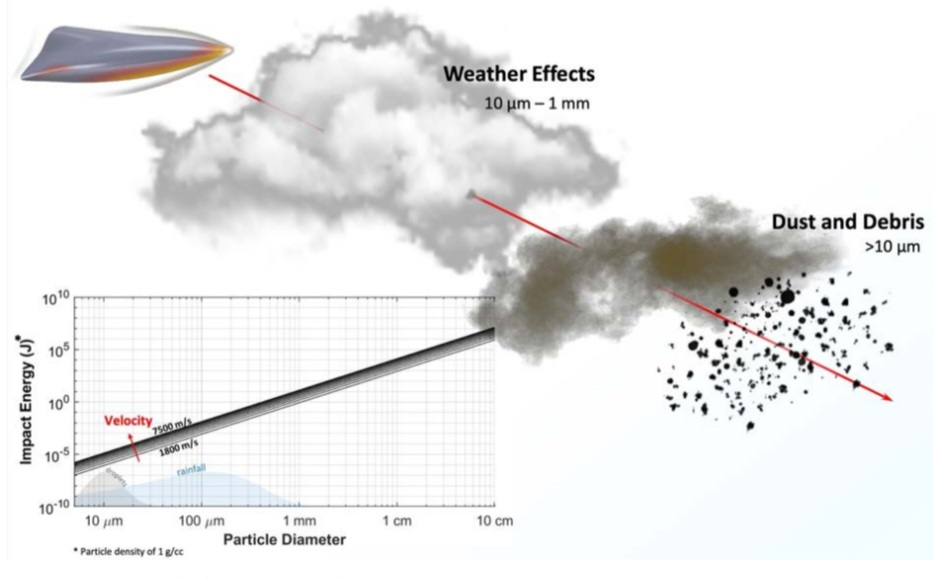
Source: Benjamin Carmichael, Southern Research. Reprinted with permission.
Directed Energy
Directed-energy systems offer another avenue for complicating hypersonic attack. Unlike kinetic effectors, directed-energy weapons may offer large magazine capacities, significantly lower cost per shot, and simpler guidance requirements. Although mostly limited by their direct line of sight, directed-energy systems may
be suited for augmenting terminal defenses or for basing close to adversary launch positions.
The prospect of using lasers for hypersonic defense has been the subject of considerable debate. Recent technical advances in continuous-wave diode-pumped solid-state, combined-fiber, diode-pumped alkali, and ultra-short pulse promise significant beam power increases with lower size, weight, and power demands. Between 2008 and 2018, demonstrated beam powers of individual fiber laser modules grew fivefold, with combined beam powers in the several-hundred-kilowatt range expected in the near term.99 Although early in development, recent research in ultrashort pulse lasers shows improved atmospheric propagation and the potential for transmitting electronic interference.100 MDA showed interest in this technology by issuing a request for information in February 2021.101
Nevertheless, even the hundred-kilowatt to megawatt-class powers expected of future laser systems may not be adequate for penetrating hypersonic thermal protection systems. Prior analyses have suggested that high-powered lasers would be unable to effectively penetrate a ballistic reentry vehicle, which possesses less thermal shielding than a hypersonic system.102 Penetrating the robust shielding of a hypersonic weapon within seconds—the time to traverse an airborne platform’s line of sight—would require beam powers significantly beyond the current state of the art.103
High-powered microwave (HPM) weapons represent another directed-energy option for hypersonic defense. HPMs are less sensitive to weather conditions than are lasers and do not require sophisticated
pointing or optical compensation systems to operate.104 Sensor data less precise than that needed for kinetic interceptor fire control could be enough to cue HPMs to “fry the sky.”
Given their considerably shorter range, HPMs may benefit from different platforms and basing modes. An earlier effort, the Air Force’s CHAMP missile, represents one approach, incorporating a miniaturized HPM payload on a cruise missile. For the hypersonic defense mission, HPMs might be deployed on loitering unmanned aircraft as a nonkinetic obstacle. Alternatively, an HPM payload could be delivered to the general vicinity of an incoming target by an interceptor booster or other platform.
High-powered microwave weapons could exploit vulnerabilities in hypersonic weapons’ communications systems and radiation shielding to achieve mission kill. Microwave radiation can enter a hypersonic weapon through antennae operating in the same frequency or through other unshielded elements of the vehicle, damaging internal electronics.105 Depending on the circuitry damaged, a microwave weapon could achieve full or partial mission kill, disrupting a vehicle’s ability to navigate, arm its warhead, or maintain level flight.
Without detailed knowledge of a weapon’s shielding, antennae, and internal circuitry, it is challenging to predict what damage an HPM weapon may cause, both to the threat and to nearby friendly assets. The uncertainty associated with HPM effects leaves them suited to early glide-phase applications, where there is more time for damage assessment and for a hypersonic weapon’s guidance errors to compound. Successful employment of HPM weapons may hinge on insights gathered from the United States’ own strategic weapons efforts and technical intelligence on foreign systems.106
Similar challenges would make it difficult to harden weapon electronics against microwave systems. Radiation can diffract through small and unintuitive entry points on a weapon, requiring extensive
testing to predict. The integration of radar guidance or navigation systems—and their associated antenna apertures—adds another layer of complication, opening vulnerabilities both to in-band and
brute-force attack. While HPM damage modeling is difficult, the United States’ decades-long industrial and intelligence advantages in these areas—combined with competitors’ reported deficiencies in
radiation-hardened circuitry—could impose costs on adversaries aiming to counter an HPM defense.107
With comparatively limited range, HPM weapons may be well suited as a complement for terminal defenses.108 Improvements in microwave weapon size, weight, and power could allow their carriage on aircraft or on long-range interceptors with loitering capability, denying volumes of airspace earlier
in a hypersonic weapon’s flight. Microwave weapons, particularly those optimized for “front-door” electronic attack, would also complement terminal defenses—especially against hypersonic and highspeed
weapons equipped with radar terminal guidance. By disrupting a hypersonic weapon’s final evasive maneuvers or seekers, a microwave weapon could ease the agility requirements for kinetic
terminal interceptors or achieve a mission kill by sending the missile off course.
Modular Payloads
A comprehensive approach to hypersonic defense might include an interceptor or other platform capable of accommodating multiple payload types, such as blast-fragmentation, particle-dispensing,
hit-to-kill, directed-energy, or electromagnetic systems. A common booster system with various warhead types would create doubt about which modalities an attacker needs to overcome, and from where (Figure 25).
Figure 25: Modular Payload Concept
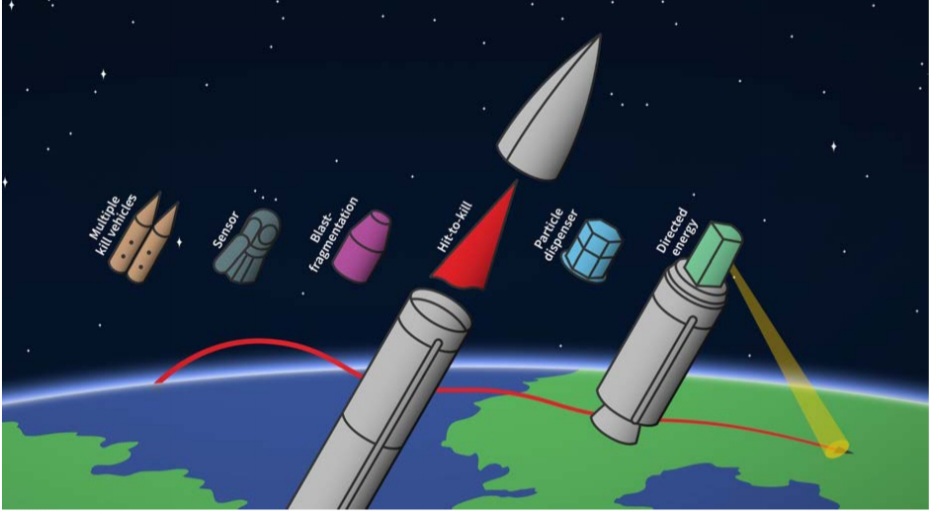
Source: CSIS Missile Defense Project.
Alternative payload types could offer greater flexibility than traditional hit-to-kill systems, creating dilemmas for adversaries. New varieties of particulate warheads, for example, could be fielded at a faster pace than new hypersonic weapons, imposing costs on an aggressor. Without the exquisite demands of “detect-control-engage,” area-wide particulate defense could positively affect the cost curve. Payloads could be developed with different densities, volumes, and pyrotechnic, incendiary, or
corrosive characteristics, with dispersion patterns optimized to defeat an evolving threat. Hardening a hypersonic weapon against marginal changes in particle characteristics could require an extensive
system engineering effort given the deep level of integration inherent in hypersonic weapon design.
Questions arise about cost and operational effectiveness of these concepts. For now, the most important efforts remain the sustainment and effective fielding of a space sensor layer and glidephase
kinetic interceptor. But the threat spectrum is not getting any less stressing. It is certain that highly agile threats will continue to proliferate, including other types of hypersonic delivery systems,
spaceplanes, and orbital bombardment. The imagination required to comprehend the hypersonic defense challenge must not be confined to hypersonic glide vehicles and hypersonic cruise missiles but
must anticipate these other types as well. In this context, the promise of area-wide effects and volume kill mechanisms will warrant sustained attention.
Reformulating the Mission
▪ The past policy approach of devising continent-wide defenses of the homeland against ballistic missile attacks is neither technically nor fiscally affordable for highly maneuverable threats, including hypersonic missiles.
▪ Countering hypersonic threats should instead emphasize the defense of a select number of critical assets, primarily abroad but also for the homeland.
▪ Active defense assets are becoming increasingly vulnerable to complex and integrated attack. To avoid suppression, passive defense principles must inform defense design.
▪ Sensors and other assets employed for active defense are a force multiplier for both passive defenses and missile defeat, and their costs can be spread over various mission sets.
▪ Hypersonic weapons strain the geographic organizing principles of combatant commands and services, crossing areas of responsibility and the boundary between air and space.
▪ To realize this adaptation, doctrinal and operational changes may be required to enable combatant commands and other entities to share information and communicate operational decisions more
quickly and at lower echelons.
The objectives of hypersonic defense will necessarily differ from the continent-wide defense against rogue state ballistic missile threats. In short, it will be impossible to defend everything, and the defended asset list must be prioritized accordingly. Given the role of hypersonic threats in near-peer orders of battle,
hypersonic defense efforts should prioritize the defense of forward-deployed forces and a limited number of critical homeland assets. The complexity and scale of the threat also requires active hypersonic defense measures to be complemented by expanded offensive capabilities and passive defense.
Preferential Defense
The defended asset list for any hypersonic defense architecture must be limited. As with cruise missile defense, it will be unaffordable and unrealistic to encircle the United States with hypersonic defense
interceptors.109 Adversaries’ likely stockpiles of hypersonic weapons, and their individual difficulty to defeat, will force difficult choices over defended areas. While this reality has always been present and understood for forward-based force protection, its application to homeland defense will require a candid reassessment of policy.
In addition to supporting strategic nuclear deterrence, peer competitors appear to emphasize the role of hypersonic weapons in winning regional conflicts and in preventing U.S. forces from accessing the
battlespace.110 The U.S. Joint Force depends on land- and carrier-based airpower in theater to enable its
regional power projection.111 Large numbers of hypersonic weapons would be used to engage critical points, such as runways, aircraft carriers, air defenses, and command and control nodes. A future
hypersonic defense might prioritize a modest number of vital locations to defend, especially those relating to logistics, command and control, and power projection.
Passive Defense and Deception
Active defense alone cannot contend with the expected volume of hypersonic, cruise, and advanced ballistic missiles. Passive defense must also play an increased role in a comprehensive approach to countering advanced hypersonic threats. Forward-deployed forces must above all frustrate adversary targeting.112 In the nearer term, existing bases could make greater use of dispersal, decoys, camouflage, and other forms of deception to confound hypersonic weapons’ terminal guidance systems—methods previously explored by U.S. adversaries.113 In the longer term, the Joint Force must continue to transition “from large, centralized, unhardened infrastructure to smaller, dispersed, resilient, adaptive basing that include active and passive defenses.”114 Critical air and missile defense nodes could also be concealed in mobile and containerized platforms, along with decoys, to complicate targeting. Operational procedures can also improve survivability. Additional investments in training for damage control and runway repair, coupled with the unpredictable rotation of forces between bases, could
mitigate the destruction or disruption of forward-deployed forces.115
The interplay of active and passive missile defenses should also receive greater attention in force planning. Passive approaches—such as the alert and subsequent dispersal of forces upon indications and warning—may well depend upon some of the same sensors employed in active defense to provide early threat assessments and predicted impact points.116 Successful early warning procedures are not only useful for cueing active missile defense. Advance indications and warning were critical to mitigating casualties before and during Iran’s January 2020 attack on U.S. soldiers at the Al Asad base in Iraq.
Active defense assets are becoming increasingly vulnerable to complex and integrated attack. To avoid suppression, passive defense principles must inform defense design.117 Active and passive defenses are not true substitutes but rather are complements, and their integration should remain a priority for designing future missile defense architectures writ large. The emergence of hypersonic missiles, and the diffusion of precision missiles more generally, merely heightens the urgency of such an approach.
Missile Defeat
Finally, countering hypersonic missiles will require greater reliance on a comprehensive approach to missile defeat—the full spectrum of offensive and defensive methods to deter adversary missile launches, including coordinated attack operations before launch.118 Cyber and electronic warfare may play a significant role in defeating hypersonic threats of all types, including far left of launch, affecting the overall cost calculus and force sizing metric for active defenses.
As with passive defenses, missile defeat benefits from the capabilities utilized in active missile defense. In addition to providing persistent global coverage, for example, the forthcoming elevated air- and
space-based hypersonic sensor constellation could aid intelligence, surveillance, and reconnaissance in locating mobile missile launchers.119 Integrating defensive sensors with offensive hypersonic strike would, in turn, help the Joint Force to promptly target adversary launchers, raising the costs
of initiating a conflict. The United States does not compete with unlimited resources. By integrating defensive sensor networks into offensive kill chains, the Joint Force can bolster missile defeat and
distribute the cost of space- and ground-based sensors across a larger mission set.120
Data, Doctrine, and Organization
All long-range air or missile threats have the potential to strain the geographic organizing principle of existing combatant commands (COCOMs). Hypersonic missiles do so differently and to a greater
degree than others. In addition to circumventing existing defense designs with their combination of altitude, trajectory, and speed, the qualities of hypersonic missiles attack the gaps and seams of U.S. and allied organizations and doctrine.
“Existing doctrine and organizational structure may not be adequate to address the cross-domain threat posed by [high-speed maneuvering weapons].”121
— National Academies of Sciences, High-Speed Maneuvering Weapons (2016)
The flight of long-range hypersonic weapons systems may traverse multiple areas of responsibility (AORs). Their speed and maneuverability reduce the time to communicate and coordinate a response
between commands. The ability of hypersonic weapons to exploit friction and lag in the U.S. command and control structure may indeed be one of their attractions for adversaries, and a qualitative difference relative to other delivery systems.
The issue has rapidly become apparent as hypersonic threats proliferate. In 2016, JIAMDO director Rear Admiral Edward Cashman observed that “The traditional definitions and threat characteristics
which have defined our capability development and organizational structures are breaking down.” A similar point was made that same year by the National Academy of Sciences: “Existing doctrine and organizational structure may not be adequate” to address emerging hypersonic threats. That study cited a “lack of leadership coordination . . . for the development of possible countermeasures” and found “no
formal strategic operational concept or organizational sense of urgency.”122
In its 2017 Joint Publication, Countering Air and Missile Threats (JP 3-01), the Joint Chiefs of Staff identified “operational seams” across geographic AORs, recognizing the nature of the threat as
“transregional, multi-domain, and multi-functional.”123 Before then, U.S. doctrine had lacked a formal authority for coordinating cross-COCOM air and missile defense. While STRATCOM assumed
a planning “synchronizer” role for ballistic missile defense in earlier iterations of JP 3-01, it did not play a similar function in planning against aerodynamic threats.124 Although the JP 3-01’s latest edition still emphasizes ballistic missile defense as the primary focus of cross-AOR planning, doctrine now recognizes the need for cross-AOR coordination for hypersonic defense.125 These revisions, while necessary, may remain insufficient for achieving an integrated, effective hypersonic defense. Combatant commands require common data platforms between existing service programs and geographic areas as well as an ability to rapidly collaborate across those platforms during a pending or
actual attack.
NORTHCOM head General Glen VanHerck has emphasized the organizational barriers to cross-COCOM information sharing for cruise missile defense, noting that joint operating pictures were only formed at higher levels of command, “taking hours, if not days” to make decisions.126 These structural challenges are underscored by the lack of available tools for communicating decisions. Short windows of detection and engagement may preclude operators from “picking up a telephone to talk to the command center,
who would then pick up another telephone [to contact the air defense sector]” before reaching command authorities “through another phone call” in several minutes.127 This problem is compounded by
hypersonic weapons’ AOR-crossing characteristics, as defensive operations must be coordinated verbally across multiple regions. Organization and doctrinal changes to address this shortcoming may require alterations to the Unified Command Plan.128 Combatant commands and the military services that provide their early warning and threat tracking sensors need common cloud-based data platforms and data sharing mandates to break down longstanding stovepipes. Gone are the days when these commands, faced with a threat, enjoy the time to make a formal request for intelligence imagery or to place a phone call to gather more information. Today, commands require common data sharing, visualization, and decisionmaking platforms to enable them to collaborate in real time from the same picture.
These common platforms should integrate into an evolved multi-threat Command, Control, Battle Management, and Communications (C2BMC) capability. Rather than maintain an exclusive focus on
ballistic missile warning, tracking, and engagement, the category-straddling hypersonic threat requires expanding the scope of C2BMC’s mission set. Integrating new and existing sensors and data sets—both government and commercial space-based sensing—into C2BMC could expand detection and tracking of both hypersonic and lower-speed cruise missile threats. Additional sensors and data pipelines, combined with machine learning-assisted interpretive tools, would expand C2BMC’s capacity to generate response options and support human decision.
International Cooperation and the Industrial Base
▪ While legacy missile defense infrastructure provides a solid foundation for hypersonic defense, additional investments in hypersonic-related workforce, testing, and manufacturing will be necessary to remain competitive.
▪ The production of offensive hypersonic systems and future glide-phase effectors are bottlenecked by a small supplier and manufacturing base for thermal protection systems, insufficient wind tunnel capacity, and too few multi-fidelity modeling capabilities to validate designs.
▪ Over the past decades, the hypersonic workforce has suffered from budgetary boom-and-bust cycles, shedding valuable talent with each successive cycle.
▪ Three problem areas—hypersonic design infrastructure, validation, and budget instability—remain the focal points for industrial base reform.
▪ Forward-deployed surface-based sensors and effectors may require political and basing accommodations. Improved sensor and effector mobility and range could mitigate these challenges.
The realization of hypersonic defense will also require an overhaul of the defense industrial base and international partnerships. While the United States can draw from an existing ballistic missile defense industrial base for many investments, reforms to the hypersonic workforce, testing capabilities, and
manufacturing are needed to support further development. The United States also continues to benefit from its allied and partner relationships in developing and deploying hypersonic systems. As America moves forward with fielding hypersonic systems, it should work with allies to ensure common standards for data transfer, basing, and other overlapping areas of interest.
Sunk costs from decades of ballistic missile defense experience and infrastructure can be leveraged
for the development of hypersonic defenses. Requisite improvements in data transmission, battle management, and sensing can draw upon the larger American workforce of information technology personnel. Many new capabilities, such as edge computing and low earth orbit (LEO) satellites, can
leverage significant developments in the commercial sector. The potential for a proliferated LEO sensor architecture, for example, benefits from non-traditional sources of satellite launch and production that have significantly reduced costs.129 New developments in small satellite launch, spearheaded by the Department of Defense’s rapid launch initiative, may also offer the potential for reconstituting orbital
assets in conflict scenarios. Most critically, the missile defense enterprise can build upon commercial developments in satellite networking and edge computing that can reduce size and power requirements.
Other industrial base challenges are unique to hypersonic applications. Scaling up the production of carbon-carbon and other thermal protection materials—specifically in the large single pieces needed for hypersonic vehicles—remains an area of concern for offensive hypersonic systems.130 Limited
supplies of carbon-carbon composites have already stymied foreign efforts to produce hypersonic glide vehicles at scale.131 The United States maintains a significantly larger composites supplier base, but a ceiling on access to carbon-carbon could constrain the production of long-range interceptors or drive demand for alternative means of thermal protection. Work must continue to accumulate production expertise and expand composites production to support the anticipated spike in demand.
The hypersonic enterprise requires enhanced software tools for validating new designs. Research has focused on the development of computationally intensive, high-fidelity codes to simulate hypersonic
phenomena, but industry lacks usable multiphysics simulation codes adapted to the cadence of practical weapons design.132 Computer modeling represents the future for hypersonic testing and
validation, but the lack of sufficient wind tunnel testing facilities has stymied the development of new codes. The shortage of facilities to model surface chemical reactions, conduct material screening,
verify thermal protection system design, and support scramjet engine testing has bottlenecked the development of more advanced models.133
Maintaining a talented workforce for offensive and defensive hypersonic systems represents a more challenging undertaking. Funding for the hypersonic enterprise has historically tended to oscillate in roughly 10-year cycles, repeatedly gaining and shedding hard-won knowledge, supply networks, and
personnel. Despite recent improvements, the engineering workforce remains too small to support the growing demand signal for hypersonic weapons. In each 10-year funding cycle since the United
States began testing boost-glide vehicles in the late 1950s, the engineering workforce has shrunk, with today’s cohort over 70 percent smaller and significantly older on average than 30 years ago.134
Especially when contrasted with the growth and stability of adversary efforts, the status quo is unsustainable for maintaining leadership in this space. Like other specialized workforces, engineers
with hypersonic experience cannot simply be hired whenever necessary; without steady funding, personnel will diffuse to other roles in the private sector and their accumulated knowledge will be lost. At congressional urging, the Joint Hypersonic Transition Office (JHTO) has created a university consortium to improve this situation, especially in applied hypersonic research, funded at about $100 million over the Future Years Defense Program.
Enhanced recruitment and management practices are necessary but not sufficient to retain talent in hypersonic programs. The software-intensive nature of these projects, combined with significant
competition from private-sector hiring, creates challenges for future talent acquisition. Some talent challenges are unrelated to compensation. Slowdowns in clearance processing, organizational
culture challenges, and inflexible project management practices can also impede efforts to cultivate a hypersonic research workforce.
In brief, the hypersonic defense industrial base benefits from existing ballistic missile defense-related supply chains but has not yet scaled key technologies common to hypersonic offense and defense, including thermal protection systems, simulation capabilities, and wind tunnel capacity. At a narrower level, the hypersonic enterprise should embrace new manufacturing technologies and workforce
management techniques to maintain leadership. Specifically, the Department of Defense should implement its workforce retention standards with greater emphasis on university collaborations and training non-degreed personnel.135 Moreover, the hypersonic enterprise should continue research into technologies that enhance the manufacturability of hypersonic systems, strike and defense alike. Recent
advances in additive manufacturing may ease the production of complex structural and propulsion system components.136 Ceramic matrix composites, meanwhile, have long been matured as a thermal
protection shielding technology, potentially offering an alternative for certain carbon-carbon parts.137
On a broader level, however, policymakers should reverse the cyclical patterns of panic and disinterest that have characterized the hypersonic enterprise. The development of hypersonic weapons is a complex undertaking, requiring a significant number of experienced personnel and deep institutional
knowledge to complete. Recent hypersonic flight test failures and mishaps may be a symptom of this shortfall. Enhanced recruitment practices are only a partial solution to the flagging hypersonic
workforce. The United States cannot afford to repeatedly discard these capabilities at a loss and overspend to acquire them again. Sustained funding and achievable timelines will be critical.
Deepened cooperation with allies could help to mitigate these shortfalls. Past partnerships on hypersonic research—such as with Australia—have produced technical breakthroughs for offensive and defensive
systems. In 2020, the United States and Australia renewed their technical cooperation through the Southern Cross Integrated Flight Research Experiment (SCIFiRE), collaborating on hypersonic basic research and flight test activity. Future cooperation with other allies could allow for similar leaps for hypersonic defense.138 Given the connectivity between strike and defense, it will be important to monitor interpretations of the Missile Technology Control Regime that could throttle international cooperation.
The utility of distributed force postures for area defense also points to the need for new political arrangements with allies. The greater numbers of forward deployed elements may also increase the demand for expanded hosting agreements. To support these postures, the United States could benefit from deeper sensor integration with allies. While the United States and its allies have achieved some
degree of integration through its European Phased Adaptive Approach and Japanese Aegis systems, the United States must broker more comprehensive tracking data sharing agreements for hypersonic
defense. In turn, the exchange of tracking data between allied militaries will require new statutory authority with consideration for operational security and foreign military sales concerns.
The political challenges associated with forward basing underscores the necessity of procuring long-range defenses. Countering hypersonic weapons in the glide phase not only presents technical
benefits but would increase time for decisionmaking and potentially reduce the number of overseas bases needed for wide-area coverage. Investments in mobile basing for both sensors and effectors—
particularly using aircraft—could also present footprint benefits for U.S. forward forces. Space-based sensor architectures would allow for even smaller footprints while maintaining greater sensor coverage.
Conclusion
A Difficult but Tractable Problem
The 2018 National Defense Strategy identified long-term strategic competition with near-peer states as the central challenge of our time. Hypersonic and other advanced missile threats represent a key problem for U.S. power projection and the military dimension of this strategic competition. As a practical matter, access to strategic theaters requires effective hypersonic defenses. Developing and
fielding such defenses will be challenging but not impossible. Hypersonic missiles are not unstoppable.
Hypersonic flight is atmospheric flight, and as such, hypersonic missile defense is a complex form of air defense. Framing the mission as complex air defense will become increasingly useful for understanding and contending with the growing diversity of threats arriving in and through the atmosphere. Recognizing that the hypersonic defense problem is more than an adjunct to legacy ballistic missile defense both points toward the scope of the problem and the potential to imaginatively exploit vulnerabilities in the hypersonic flight regime.
An effective hypersonic defense must include space sensors and a glide-phase interceptor, but it should not stop there. Hypersonic weapons are not silver bullets, and neither will there be a single, silver-bullet solution. Numerous efforts pursued in tandem across a comprehensive architecture will be necessary to meet the challenge. A more limited defended asset list, passive defense, attack operations, and other means to channel the threat can inform a realistic defense design and permit affordable force sizing metrics. Different approaches to “layering” defenses will force adversaries to harden against a variety of physical and electromagnetic phenomena. Alternative kill mechanisms and area-wide effectors would not only constrain an adversary’s ability to plan attacks but also strain the cadence of adversary weapon design. Hypersonic weapons do not follow predictable trajectories. The United States likewise cannot afford to be predictable in its response.
Addressing the hypersonic missile threat should be the key driver to rethinking missile defense and defeat, and for transforming the legacy Ballistic Missile Defense System into the Missile Defense
System. Such a transition would not only support the hypersonic defense mission but lay the groundwork for detecting and defeating the increasingly broad missile threat spectrum, to include cruise missiles, spaceplanes, and orbital bombardment. Inasmuch as the characteristics of hypersonic missiles may come to define the future of missile warfare, giving up on active hypersonic defense could
ultimately lead to capitulation for active missile defense missions more broadly. How the hypersonic defense mission is pursued will shape the future of the missile defense enterprise.
About the Authors
Tom Karako is a senior fellow with the International Security Program and the director of the Missile Defense Project at the Center for Strategic and International Studies (CSIS), where he arrived in
His research focuses on national security, missile defense, nuclear deterrence, and public law. In 2010–2011, he was an American Political Science Association congressional fellow, working with the professional staff of the House Armed Services Committee and the Subcommittee on Strategic Forces on U.S. strategic forces policy, nonproliferation, and NATO. Dr. Karako is also currently a fellow with the Institute for Politics and Strategy of Carnegie Mellon University. He received his PhD from Claremont Graduate University and his BA from the University of Dallas.
Masao Dahlgren is a research associate with the Missile Defense Project at the Center for Strategic and International Studies (CSIS), where he focuses on deterrence and emerging technologies. Prior to joining CSIS, he worked at the Middlebury Institute of International Studies and the U.S. Department of Justice. Masao graduated summa cum laude from American University with a BA in international studies.
Endnotes
1 NATO Science and Technology Organization, Science and Technology Trends 2020-2040: Exploring the S&T
Edge (Brussels: NATO, 2020), https://www.nato.int/nato_static_fl2014/assets/pdf/2020/4/pdf/190422-ST_
Tech_Trends_Report_2020-2040.pdf; Kelley Sayler, Hypersonic Weapons: Background and Issues for Congress, CRS Report No. R45811 (Washington, DC: Congressional Research Service, 2020), https://sgp.fas.org/crs/ weapons/R45811.pdf; Demetri Sevastopulo and Kathrin Hille, “China Tests New Space Capability with Hypersonic Missile,” Financial Times, October 16, 2021, https://www.ft.com/content/ba0a3cde-719b-4040-93cb-a486e1f843fb; and Office of the Secretary of Defense, Military and Security Developments Involving the
People’s Republic of China 2021, Annual Report to Congress (Washington, DC: U.S. Department of Defense,
2021), https://media.defense.gov/2021/Nov/03/2002885874/-1/-1/0/2021-CMPR-FINAL.PDF.
2 The White House, Interim National Security Strategic Guidance (Washington, DC: March 2021), https://www.
whitehouse.gov/wp-content/uploads/2021/03/NSC-1v2.pdf.
3 Advance Policy Questions for Lloyd J. Austin, Nominee for Appointment to Be Secretary of Defense, 117th Cong.,
1st sess., January 19, 2021 (statement of Lloyd J. Austin, Nominee for Secretary of Defense), https://www.armed-services.senate.gov/imo/media/doc/Austin_APQs_01-19-21.pdf.
4 Advance Policy Questions for Dr. Kathleen Hicks, Nominee for Appointment to Be Deputy Secretary of Defense,
117th Cong., 1st sess., February 2, 2021 (statement of Kathleen Hicks, Nominee for Deputy Secretary of Defense),https://www.armed-services.senate.gov/imo/media/doc/Hicks_APQs_02-02-21.pdf.
5 David H. Berger, Commandant’s Planning Guidance (Washington, DC: U.S. Marine Corps, 2019), https://www.hqmc.marines.mil/Portals/142/Docs/%2038th%20Commandant%27s%20Planning%20Guidance_2019.pdf?ver=2019-07-16-200152-700; and Thomas Karako, “Seven Questions for the Future of
Air and Missile Defense” (presentation, Space and Missile Defense Symposium, Huntsville, AL, August 10, 2021).
6 U.S. Department of Defense, Missile Defense Review (Washington, DC: U.S. Department of Defense, 2019), https://media.defense.gov/2019/Jan/17/2002080666/-1/-1/1/2019-MISSILE-DEFENSE-REVIEW.PDF.
7 As an expression of velocity, Mach numbers are themselves relative figures, adjusted for the changing
density and other characteristics of air at different altitudes and temperatures. For instance, Mach 10
represents a 2.95 km/s true velocity at 20 km altitude but 3.15 km/s at 60 km altitude. Hypersonic
flight conditions do not occur at a specific absolute velocity, in km/s, occurring at different absolute
speeds depending on the characteristics of the surrounding air. NOAA, U.S. Standard Atmosphere, 1976
(Washington, DC: NOAA, 1976), https://www.ngdc.noaa.gov/stp/space-weather/online-publications/
miscellaneous/us-standard-atmosphere-1976/us-standard-atmosphere_st76-1562_noaa.pdf.
8 Michael White, “Hypersonic Strike and Defense” (speech, Center for Strategic and International Studies,
June 2, 2021), https://www.csis.org/events/hypersonic-strike-and-defense.
9 HGVs are alternatively termed “boost-glide” vehicles. Hypersonic vehicles generate lift both from their
basic shape and from the shockwave generated from their extreme velocity. The principle of using the
vehicle’s shockwave to produce lift is termed “wave-riding” and is used by a wide variety of air-breathing
and boost-glide vehicles.
10 Dean Wilkening, “Hypersonic Weapons and Strategic Stability,” Survival 61, no. 5 (2019): 129–48, doi:10.
1080/00396338.2019.1662125; John A. Tirpak, “The Hypersonics Push,” Air Force Magazine, April 1, 2020,
https://www.airforcemag.com/article/the-hypersonics-push/; and Ronald P. Fuchs et al., Why and Whither
Hypersonics Research in the US Air Force, SAB-TR-00-03 (Washington, DC: U.S. Air Force Scientific Advisory
Board, 2000), https://apps.dtic.mil/sti/citations/ADA387782.
11 Tirpak, “The Hypersonics Push.”
12 White, “Hypersonic Strike and Defense”; and Ronald S. Fry, “A Century of Ramjet Propulsion Technology
Evolution,” Journal of Propulsion and Power 20, no. 1 (2004), 27–58, doi:10.2514/1.9178.
13 Demetri Sevastopulo, “Chinese hypersonic weapon fired a missile over South China Sea,” Financial Times,
November 21, 2021, https://www.ft.com/content/a127f6de-f7b1-459e-b7ae-c14ed6a9198c; and Demetri
Sevastopulo, “China conducted two hypersonic weapons tests this summer,” Financial Times, October 20,
2021, https://www.ft.com/content/c7139a23-1271-43ae-975b-9b632330130b.
14 Loveneesh Rana and Bernd Chudoba, “Design Evolution and AHP-Based Historiography of Lifting
Reentry Vehicle Space Programs,” in American Institute of Aeronautics and Astronautics, AIAA SPACE
2016 (Long Beach, CA: 2016), doi:10.2514/6.2016-5319; and National Research Council, U.S. Conventional
Prompt Global Strike: Issues for 2008 and Beyond (Washington, DC: National Academies Press, 2008),
doi:10.17226/12061.
15 See, for instance, Steven T. Dunham and Robert S. Wilson, The Missile Threat: A Taxonomy for Moving
Beyond Just Ballistic (Washington, DC: Aerospace Corporation, 2020), https://aerospace.org/sites/default/
files/2020-08/Wilson-Dunham_MissileThreat_20200826_0.pdf.
16 This insight and formulation about air defense comes from Rear Admiral Archer Macy, USN retired.
17 John D. Anderson Jr., Hypersonic and High-Temperature Gas Dynamics, 3rd ed. (Reston, VA: American
Institute of Aeronautics and Astronautics, 2019); John J. Bertin and Russell M. Cummings, “Critical
Hypersonic Aerothermodynamic Phenomena,” Annual Review of Fluid Mechanics 38, no. 1 (2006): 129–57,
doi:10.1146/annurev.fluid.38.050304.092041; and Kei Y. Lau, “Aerothermodynamics and Hypersonic
Vehicle Design” (presentation, Fundamentals of Hypersonic Flight Short Courses, Online, August 17,
2016), https://www.slideshare.net/KeiYunlau/2016aerothermallaurevb.
18 John J. Bertin, Hypersonic Aerothermodynamics, AIAA Education Series (Reston, VA: American Institute of
Aeronautics and Astronautics, 1994); and Terry H. Phillips, A Common Aero Vehicle (CAV) Model, Description,
and Employment Guide (Washington, DC: Schafer Corporation, 2003).
19 Kevin G. Bowcutt, “Tackling the Extreme Challenges of Air-Breathing Hypersonic Vehicle Design,
Technology, and Flight” (presentation, Mathematics, Computing and Design Symposium, Stanford, CA,
November 21, 2014), http://aero-comlab.stanford.edu/jameson/aj80th/bowcutt.pdf.
Anderson, Hypersonic and High-Temperature Gas Dynamics; National Research Council, “Technologies
Relevant to Hypersonic Vehicles and Their Status,” in Hypersonic Technology for Military Application
(Washington, DC: National Academies Press, 1989), 13–56.
21 David E. Glass, “Hypersonic Materials and Structures” (presentation, Society for the Advancement of
Material and Process Engineering (SAMPE) Conference, Baltimore, MD, May 18, 2015); C. R. McClinton et
al., “Airbreathing Hypersonic Technology Vision Vehicles and Development Dreams,” in 9th International
Space Planes and Hypersonic Systems and Technologies Conference, AIAA No. 99-4978 (Norfolk, VA: American
Institute of Aeronautics and Astronautics, 1999), doi:10.2514/6.1999-4978; and Garrett Reim, “7 Technical
Challenges That Need to Be Overcome by Hypersonic Missile Builders,” Flight International, May 7, 20220,
https://www.flightglobal.com/flight-international/7-technical-challenges-that-need-to-be-overcome-byhypersonic-missile-builders/138237.article.
22 White and Price, “Affordable Hypersonic Missiles for Long-Range Precision Strike.”
23 Philip E. Ross, “Russia, China, the U.S.: Who Will Win The Hypersonic Arms Race?,” IEEE Spectrum,
November 17, 2020, https://spectrum.ieee.org/russia-china-the-us-who-will-win-the-hypersonic-armsrace#toggle-gdpr;
and Philip Ligrani, “Traditional Heat Transfer and Fluid Mechanics Investigations for
Defensive Hypersonic Activities” (presentation, IDGA Counter Hypersonic Weapons Summit, Online, July
24, 2020).
24 Eric Marineau, “ONR Foundational Research Supporting Defensive Hypersonic Efforts” (presentation,
IDGA Counter Hypersonic Weapons Summit, Online, July 24, 2020); Philip Ligrani, “R&D in Experimental
Diagnostics: High Speed Flows and Air Breathing Propulsion Materials” (presentation, Autonomous and
Hypersonic Weapons Systems Virtual Symposium, Online, November 5, 2020); Russell M. Cummings
et al., “Advancing Challenging Aerothermodynamic and Propulsion System Phenomena via Hypersonic
Vehicle Simulation” (presentation, IDGA Hypersonic Weapons Summit, Online, March 31, 2020),
https://player.vimeo.com/video/471480938; Manuel D. Salas, “A Review of Hypersonics Aerodynamics,
Aerothermodynamics and Plasmadynamics Activities Within NASA’s Fundamental Aeronautics Program,”
in 39th AIAA Thermophysics Conference, AIAA No. 2007-4264 (Miami, FL: American Institute of Aeronautics
and Astronautics, 2007), doi:10.2514/6.2007-4264; and Mark J. Lewis, “Hypersonic Flight: A Status Report”
(presentation, NDIA Hypersonics Capabilities Conference, West Lafayette, IN, July 30, 2019), https://ndia.
dtic.mil/2019/2019hypersonics.html.
25 Bertin, Hypersonic Aerothermodynamics; Katya M. Casper et al., “Hypersonic Fluid-Structure Interactions
on a Slender Cone,” in 2018 AIAA Aerospace Sciences Meeting, 0 vols., AIAA SciTech Forum (Reston, VA:
American Institute of Aeronautics and Astronautics, 2018), doi:10.2514/6.2018-1825; Scott A. Berry and
Thomas J. Horvath, “Discrete-Roughness Transition for Hypersonic Flight Vehicles,” Journal of Spacecraft
and Rockets 45, no. 2 (March 2008): 216–27, doi:10.2514/1.30970; Meelan M. Choudhari, Fei Li, and
Pedro Paredes, “Effect of Distributed Patch of Smooth Roughness Elements on Transition in a High-Speed
Boundary Layer,” in 2018 Fluid Dynamics Conference (presentation, 2018 Fluid Dynamics Conference,
Atlanta, Georgia: American Institute of Aeronautics and Astronautics, 2018), doi:10.2514/6.2018-3532.
26 Jeffrey Dickeson, “Advancements in Hypersonic Weapons Systems and Capabilities” (presentation, TTC
Military Hypersonic Weapon Systems Conference, Online, October 18, 2021).
27 Ibid; Sarah Popkin, “AFOSR Hypersonic Basic Research” (presentation, TTC Military Hypersonic Weapon
Systems Conference, Online, October 18, 2021); Ligrani, “Traditional Heat Transfer and Fluid Mechanics
Investigations for Defensive Hypersonic Activities”; Marineau, “ONR Foundational Research Supporting
Defensive Hypersonic Efforts”; Anderson, Hypersonic and High-Temperature Gas Dynamics; and Christoph
Hader, “Laminar-Turbulent Boundary-Layer Transition on a Flared Cone at Mach 6” (presentation,
Advanced NASA Modeling & Simulation (AMS) Seminar Series, Moffett Field, CA, May 11, 2017), https://
www.nas.nasa.gov/publications/ams/2017/05-11-17.html.
28 Vishal Giare, “Developing a Comprehensive Approach for Hypersonic Threat Defeat” (presentation, IDGA
Hypersonic Weapons Summit, Online, April 29, 2021).
Thomas Karako and Wes Rumbaugh, Distributed Defense: New Operational Concepts for Integrated Air and
Missile Defense (Washington, DC: Center for Strategic and International Studies, 2018), https://csiswebsite-prod.s3.amazonaws.com/s3fs-public/publication/171206_Karako_DistributedDefense_Web_0.pdf;
Thomas Karako, “Beyond the Radar Archipelago: A New Roadmap for Missile Defense Sensors,” War on the
Rocks, November 28, 2018, https://warontherocks.com/2018/11/beyond-the-radar-archipelago-a-newroadmap-for-missile-defense-sensors/.
30 Brian Lihani, “Advancing Aerospace Warning of Hypersonic Threats” (presentation, IDGA Counter
Hypersonic Weapons Summit, Online, July 23, 2020); and Dennis M. Gormley, Missile Contagion: Cruise
Missile Proliferation and the Threat to International Security (Annapolis, MD: Naval Institute Press, 2010).
31 Steven Simon, “Hypersonic Missiles Are a Game Changer,” New York Times, January 2, 2020, https://www.
nytimes.com/2020/01/02/opinion/hypersonic-missiles.html.
32 “National Defense Authorization Act for Fiscal Year 2017,” Pub. L. No. 114–328, § 1686-1687, 130 Stat.
2628-2630 (2016).
33 Thomas Karako, “Trump’s Blind Spot,” Politico, September 6, 2019, https://politi.co/2zVtaIS; and Melanie
Marlowe, “Three Obstacles Are Slowing Space Sensors for Hypersonic Threats,” C4ISRNet, April 8, 2020,
https://www.c4isrnet.com/space/2020/04/08/three-obstacles-are-slowing-space-sensors-for-hypersonicthreats/.
34 Sandra Erwin, “Next Steps for the Pentagon’s New Space Sensors for Missile Defense,” SpaceNews, January
21, 2019, https://spacenews.com/next-steps-for-the-pentagons-new-space-sensors-for-missile-defense/.
35 Sandra Erwin, “DoD Space Agency to Award Multiple Contracts for up to 150 Satellites,” SpaceNews, March
4, 2021, https://spacenews.com/dod-space-agency-to-award-multiple-contracts-for-up-to-150-satellites/;
and Roger Teague, “SBIRS Transformational Capability,” U.S. Air Force, Space and Missile Systems Center,
November 30, 2006, https://nsarchive2.gwu.edu/NSAEBB/NSAEBB235/new54.pdf.
36 Cameron L. Tracy and David Wright, “Modeling the Performance of Hypersonic Boost-Glide Missiles,”
Science & Global Security 28, no. 3 (September 1, 2020), 135–70, doi:10.1080/08929882.2020.1864945; and
Michael D. Griffin, “Ensuring U.S. Technological Superiority: An Update from Under Secretary Michael
D. Griffin” (speech, Hudson Institute, Washington, D.C., August 13, 2019), https://s3.amazonaws.com/
media.hudson.org/Transcript%20–%20An%20Update%20from%20Under%20Secretary%20Michael%20
Griffin2.pdf.
37 Qinglin Niu et al., “Infrared Radiation Characteristics of a Hypersonic Vehicle under Time-Varying Angles
of Attack,” Chinese Journal of Aeronautics 32, no. 4 (April 2019): 861–74, doi:10.1016/j.cja.2019.01.003; and
Missile Defense Agency, FTM-20 in the Ballistic Missile Defense System, 13-MDA-7168 (Washington, DC:
2013), https://www.mda.mil/global/documents/pdf/Aegis_FTM-20_FactSheet.pdf.
38 C. Todd Lopez, “Agency Awards Contracts for Tracking Layer of National Defense Space Architecture,”
U.S. Department of Defense, October 5, 2020, https://www.defense.gov/News/News-Stories/Article/
Article/2372647/agency-awards-contracts-for-tracking-layer-of-national-defense-space-architectu/.
39 Jon Hill, in “2021 McAleese Defense Programs Conference Speaker: Vice Admiral John A. Hill,”
YouTube Video, posted by McAleese and Associates, May 19, 2021, 48:38, https://www.youtube.com/
watch?v=5L9yufZe57Y.
40 Ibid.
41 Thomas Karako and Wes Rumbaugh, Inflection Point: Missile Defense and Defeat in the 2021 Budget
(Washington, DC: Center for Strategic and International Studies, 2020), https://www.csis.org/analysis/
inflection-point-missile-defense-and-defeat-2021-budget.
42 “National Defense Authorization Act for Fiscal Year 2021,” Pub. L. No. 116–283, § 1645 (2021), https://
www.congress.gov/116/bills/hr6395/BILLS-116hr6395enr.pdf.
Ibid.
44 Erwin, “DoD Space Agency to Award Multiple Contracts for up to 150 Satellites”; and Derek Tournear,
“Space Development Agency: Delivering Capabilities” (presentation, Smallsat Symposium, Online,
February 11, 2021), https://www.sda.mil/direct-from-smallsat-symposium-dr-derek-m-tournear-directorspace-development-agency-keynote-address/.
45 Office of the Under Secretary of Defense (Comptroller), Department of Defense Fiscal Year (FY) 2022 Budget
Estimates: Space Development Agency Research, Development, Test and Evaluation, Defense-Wide (Washington,
DC: U.S. Department of Defense, 2021), https://comptroller.defense.gov/Portals/45/Documents/
defbudget/fy2022/budget_justification/pdfs/03_RDT_and_E/SDA_PB2022_v2.pdf.
46 Harry W. Jones, “The Recent Large Reduction in Space Launch Cost,” in 48th International Conference
on Environmental Systems, ICES-2018-81 (Albuquerque, NM, 2018), https://ttu-ir.tdl.org/bitstream/
handle/2346/74082/ICES_2018_81.pdf; Philip Stockdale and Scott Aughenbaugh, “Low-Cost Access to
Space: Military Opportunities and Challenges,” Defense Horizons 83 (February 2018), https://inss.ndu.edu/
Portals/68/Documents/defensehorizon/DH-83.pdf; Air Force Space Command, The Future of Space 2060
and Implications for U.S. Strategy: Report on the Space Futures Workshop (Washington, DC: U.S. Department of
Defense, 2019), https://aerospace.csis.org/wp-content/uploads/2019/09/Future-of-Space-2060-v2-5-Sep.
pdf; and Todd Harrison et al., Implications of Ultra-Low-Cost Access to Space (Washington, DC: Center for
Strategic and International Studies, 2017), https://www.csis.org/analysis/implications-ultra-low-costaccess-space.
47 Bradley Denby and Brandon Lucia, “Orbital Edge Computing: Nanosatellite Constellations as a New
Class of Computer System,” in Proceedings of the Twenty-Fifth International Conference on Architectural
Support for Programming Languages and Operating Systems (ASPLOS ’20: Architectural Support for
Programming Languages and Operating Systems, Lausanne Switzerland: ACM, 2020), 939–54,
doi:10.1145/3373376.3378473; Ramish Zafar, “SpaceX Successfully Tests Inter-Satellite Starlink
Connectivity Via Lasers,” WCCFTech, September 3, 2020, https://wccftech.com/spacex-starlink-satellitelaser-test/;
Sandra Erwin, “DoD to Test Laser Communications Terminals in Low Earth Orbit,” SpaceNews,
June 8, 2020, https://spacenews.com/dod-to-test-laser-communications-terminals-in-low-earth-orbit/;
and Sally Cole, “Nanosats Put AI-at-the-Edge Computing to the Test in Space – Military Embedded
Systems,” Military Embedded Systems, November 16, 2020, http://militaryembedded.com/ai/machinelearning/nanosats-put-ai-at-the-edge-computing-to-the-test-in-space.
48 Wilkening, “Hypersonic Weapons and Strategic Stability.”
49 Ibid.; and Mike White and Walter R. Price, “Affordable Hypersonic Missiles for Long-Range Precision
Strike,” Johns Hopkins APL Technical Digest 20, no. 3 (1999): 415–23, https://www.jhuapl.edu/content/
techdigest/pdf/V20-N03/20-03-White.pdf.
50 Paul Zarchan, “Ballistic Missile Defense Guidance and Control Issues,” Science & Global Security 8, no. 1
(1999), 99–124, doi:10.1080/08929889908426470.
51 Jon Hill, FY21 Priorities for Missile Defense and Missile Defeat Programs, 116th Cong., 2nd sess., March 12,
2020 (statement of Jon Hill, Missile Defense Agency director), https://www.congress.gov/116/meeting/
house/110671/witnesses/HHRG-116-AS29-Wstate-HillJ-20200312.pdf.
52 Jon Hill, “MDA’s Focus on the Future” (presentation, Space and Missile Defense Symposium, Huntsville,
AL, August 12, 2021).
53 Jon Hill, “Breaking Barriers: The Next Years in Strategic Deterrence and Missile Defense” (speech, Defense
One Technology Summit, Online, June 18, 2020), https://www.dvidshub.net/video/756584/breakingbarriers-next-years-strategic-deterrence-and-missile-defense.
54 William J. Tropf et al., “Performance of Optical Sensors in Hypersonic Flight,” Johns Hopkins APL Technical
Digest 8, no. 4 (1987); Michael S. Holden, “Aerothermal and Propulsion Ground Testing That Can Be Conducted to Increase Chances for Successful Hypervelocity Flight Experiments,” in Flight Experiments for
Hypersonic Vehicle Development, RTO-EN-AVT-130, Paper 1 (Neuilly-sur-Seine: NATO RTO, 2007), 1–36;
Ligrani, “R&D in Experimental Diagnostics: High Speed Flows and Air Breathing Propulsion Materials”; and
Donald D. Duncan et al., “Experimental and Theoretical Assessment of Mechanical and Optical Effects in
Nonuniformly Heated IR Windows,” Johns Hopkins APL Technical Digest 22, no. 3 (2001): 394–408.
55 Director of Operational Test and Evaluation, FY 2017 Annual Report (Washington, DC: U.S. Department
of Defense, 2018), https://www.dote.osd.mil/Portals/97/pub/reports/FY2017/navy/2017sm-6.
pdf?ver=2019-08-19-113709-553.
56 John D. Sawyer, Missile Defense: Fiscal Year 2020 Delivery and Testing Progressed, but Annual Goals Unmet,
GAO 21-314 (Washington, DC: Government Accountability Office, 2021), https://www.gao.gov/products/
gao-21-314.
57 Samuel A. Greaves, Missile Defense Policies and Programs, 116th Cong., 1st sess., April 3, 2019 (statement of
Samuel A. Greaves, Missile Defense Agency director), https://www.armed-services.senate.gov/imo/media/
doc/Greaves_04-03-19.pdf.
58 Hill, FY21 Priorities for Missile Defense and Missile Defeat Programs,18.
59 Barbara McQuiston, Department of Defense Innovation and Research, 117th Cong., 1st sess., April 13, 2021
(statement of Barbara McQuiston, Acting Under Secretary of Defense for Research and Engineering),
https://www.appropriations.senate.gov/imo/media/doc/McQuiston%20Statement%20for%20the%20
Record.pdf.
60 Michael Griffin, “Regaining the Strategic Advantage in an Age of Great Power Competition: A Conversation
with Michael Griffin” (interview, The Hudson Institute, Washington, DC, April 13, 2018).
61 Sayler, Hypersonic Weapons: Background and Issues for Congress.
62 Jon Hill, “MDA Update” (presentation, Space and Missile Defense Symposium, Huntsville, AL, August 4,
2020).
63 Jerome Dunn, “Speed, Precision, and the Increasing Risk to Time-Sensitive Targets” (presentation, RUSI
Missile Defence and Space Conference, Online, February 26, 2020).
64 Kelley Sayler and Stephen McCall, Hypersonic Missile Defense: Issues for Congress, CRS In Focus No. IF10541
(Washington, DC: Congressional Research Service, 2021), https://crsreports.congress.gov/product/pdf/
IF/IF11623; and Missile Defense Agency, Draft Request for Prototype Proposal: Hypersonic Defense Regional
Glide Phase Weapon System, Notice No. HQ854-20-DV-OTA-01 (Huntsville, AL: Missile Defense Agency,
2020), https://sam.gov/opp/80d013d87be54757a005e90af6d22c54/view?keywords=rgpws&sort=-
relevance&index=&is_active=true&page=1.
65 Jen Judson, “MDA Pauses Defensive Hypersonic Missile Design to Refocus Plan,” Defense News, August
4, 2020, https://www.defensenews.com/digital-show-dailies/smd/2020/08/04/mda-pauses-defensivehypersonic-missile-design-effort-to-refocus-plan/.
66 White, “Hypersonic Strike and Defense.”
67 Office of the Under Secretary of Defense (Comptroller), Department of Defense Fiscal Year (FY) 2022 Budget
Estimates: Missile Defense Agency Research, Development, Test and Evaluation, Defense-Wide (Washington, DC:
U.S. Department of Defense, 2021), https://comptroller.defense.gov/Portals/45/Documents/defbudget/
fy2022/budget_justification/pdfs/03_RDT_and_E/RDTE_Vol2_MDA_RDTE_PB22_Justification_Book.pdf.
68 Jen Judson, “Here are the three companies selected to design hypersonic missile interceptors for MDA,”
Defense News, November 20, 2021, https://www.defensenews.com/pentagon/2021/11/20/heres-the-threecompanies-selected-to-design-hypersonic-missile-interceptors-for-mda/;
and Judson, “Missile Defense
Agency Dials up the Speed in Quest for Hypersonic Interceptor.
Hill, “MDA’s Focus on the Future.”
70 Missile Defense Agency, MDA Special Topic BAA HQ0851-21-S-0001: Enhanced Hypersonic Defense, Notice No.
HQ0851-21-S-0001 (Dahlgren, VA: Missile Defense Agency Sea-Based Weapon Systems Program Executive
Office, 2021), https://sam.gov/opp/8184530edf134373bc978ed0c05833e1/view; and “GPI Scenario
Animation,” YouTube video, posted by Missile Defense Agency, June 16, 2021, 7:51, https://www.youtube.
com/watch?v=-q-ieXZgrhY.
71 Office of the Under Secretary of Defense (Comptroller), Department of Defense Fiscal Year (FY) 2020
Budget Estimates: Defense Advanced Research Projects Agency Research, Development, Test & Evaluation,
Defense-Wide (Washington, DC: U.S. Department of Defense, 2019), https://comptroller.defense.gov/
Portals/45/Documents/defbudget/fy2020/budget_justification/pdfs/03_RDT_and_E/RDTE_Vol1_DARPA_
MasterJustificationBook_PB_2020.pdf; Office of the Under Secretary of Defense (Comptroller), Department
of Defense Fiscal Year (FY) 2021 Budget Estimates: Defense Advanced Research Projects Agency Research,
Development, Test & Evaluation, Defense-Wide (Washington, DC: U.S. Department of Defense, 2020), https://
comptroller.defense.gov/Portals/45/Documents/defbudget/fy2021/budget_justification/pdfs/03_RDT_
and_E/RDTE_Vol1_DARPA_MasterJustificationBook_PB_2021.pdf; and Office of the Under Secretary of
Defense (Comptroller), Department of Defense Fiscal Year (FY) 2022 Budget Estimates: Defense Advanced
Research Projects Agency Research, Development, Test & Evaluation, Defense-Wide (Washington, DC: U.S.
Department of Defense, 2021), https://comptroller.defense.gov/Portals/45/Documents/defbudget/fy2022/
budget_justification/pdfs/03_RDT_and_E/RDTE_Vol1_DARPA_MasterJustificationBook_PB_2022.pdf.
72 Hill, “Breaking Barriers.”
73 Ibid.
74 Ibid.
75 Jen Judson, “Missile Defense Agency Dials up the Speed in Quest for Hypersonic Interceptor,” Defense
News, August 13, 2021, https://www.defensenews.com/digital-show-dailies/smd/2021/08/13/missiledefense-agency-dials-up-the-speed-in-quest-for-hypersonic-interceptor/.
76 Glen VanHerck, “New Tools to Create Time and Information: ‘Building the Bike While We Ride It’,” War on
the Rocks, July 6, 2021, https://warontherocks.com/2021/07/new-tools-to-create-time-and-informationbuilding-the-bike-while-we-ride-it/.
77 Glen VanHerck, “Rethinking Homeland Defense: Global Integration, Domain Awareness, Information
Dominance and Decision Superiority” (speech, Center for Strategic and International Studies, Washington,
DC, August 17, 2021), https://www.csis.org/events/rethinking-homeland-defense-domain-awarenessinformation-dominance-and-decision-superiority.
78 Wes Rumbaugh and Thomas Karako, Seeking Alignment: Missile Defense and Defeat in the 2022 Budget
(Washington, DC: Center for Strategic and International Studies, 2021), https://www.csis.org/analysis/
seeking-alignment-missile-defense-and-defeat-2022-budget.
79 Missile Defense Agency, Report on Unfunded Priorities of the Missile Defense Agency (Washington, DC:
Government Publishing Office, 2019).
80 Jim Garamone, “Missile Defense Chief Looks to Handle Changing Threat,” U.S. Department of Defense,
June 30, 2020, https://www.defense.gov/News/News-Stories/Article/Article/2243187/missile-defensechief-looks-to-handle-changing-threat/.
81 Hill, “MDA’s Focus on the Future”; and Glen VanHerck, “Rethinking Homeland Defense.”
82 Franklin J. Hillson, “Barrage Balloons for Low-Level Defense,” Aerospace Power Journal 3, no. 2 (Summer
1989), https://web.archive.org/web/20070812023821/http://www.airpower.maxwell.af.mil/airchronicles/
apj/apj89/sum89/hillson.html.
83 National Research Council, Naval Forces’ Capability for Theater Missile Defense (Washington, DC: National Academies Press, 2001), 49, doi:10.17226/10105.
84 Tom Rowden, “Navy and Marines Demonstrate Integrated Fire Control,” U.S. Navy Commander’s Corner, Fall
2016, https://www.public.navy.mil/surfor/Pages/Navy-and-Marines-Demonstrate-Integrated-Fire-Control.
aspx.
85 Steve Trimble, “The Weekly Debrief: Air-Launched, SM-6-like Missile Exposed In New Test Photo,” Aviation
Week, April 19, 2021, https://aviationweek.com/defense-space/missile-defense-weapons/weekly-debriefair-launched-sm-6-missile-exposed-new-test.
86 CSIS Missile Defense Project, “In IBCS Test, Patriot, F-35s Intercept Cruise Missile Target,” CSIS, Missile
Threat, July 15, 2021, https://missilethreat.csis.org/in-ibcs-test-patriot-f-35s-intercept-cruise-missiletarget/.
87 National Research Council, Naval Forces’ Capability for Theater Missile Defense; and David K. Barton et al.,
“Report of the American Physical Society Study Group on Boost-Phase Intercept Systems for National
Missile Defense: Scientific and Technical Issues,” Reviews of Modern Physics 76, no. 3 (October 5, 2004):
S1–424, doi:10.1103/RevModPhys.76.S1.
88 Dean A. Wilkening, “Airborne Boost-Phase Ballistic Missile Defense,” Science & Global Security 12, no. 1–2
(January 2004), 1–67, doi:10.1080/08929880490464649; and National Research Council, Making Sense
of Ballistic Missile Defense: An Assessment of Concepts and Systems for U.S. Boost-Phase Missile Defense in
Comparison to Other Alternatives (Washington, DC: National Academies Press, 2012), doi:10.17226/13189.
89 Ibid.
90 Karako and Rumbaugh, Distributed Defense.
91 Hill, “Breaking Barriers.”
92 Michael White, “DoD Hypersonic Development Needs and Requirements” (presentation, TTC Military
Hypersonic Weapon Systems Conference, Online, October 19, 2021).
93 Marineau, “ONR Foundational Research Supporting Defensive Hypersonic Efforts”; and Ligrani,
“Traditional Heat Transfer and Fluid Mechanics Investigations for Defensive Hypersonic Activities.”
94 Giare, “Developing a Comprehensive Approach for Hypersonic Threat Defeat.”
95 B. Carmichael and S. Massey, “An Analysis of the Hypervelocity Impact Response of Graphite”
(presentation, 11th Ablation Workshop, Minneapolis, MN, September 2019); John B. Walsh, Walter
B. La Berge, and Alton D. Slay, Research, Development, Test, and Evaluation, 94th Cong., 2nd. sess., 1976
(statements of John B. Walsh, DDR&E Strategic and Space Systems deputy director, Walter B. La Berge,
Assistant Secretary of the Air Force (R&D), Alton D. Slay, R&D Deputy Chief of Staff), 6448–6529;
Matthew Bunn, “Technology of Ballistic Missile Reentry Vehicles,” in Review of U.S. Military Research and
Development 1984, eds. Kosta Tsipis and Penny Janeway (McLean, VA: Pergamon-Brassey’s International
Defense Publishers, 1984); M. L. Finson et al., Advanced Reentry Aeromechanics Final Report, ADA052744
(Andover, MA: Physical Sciences Inc., 1978), https://apps.dtic.mil/sti/citations/ADA052744; Alton D. Slay,
Air Force Research, Development, Test, and Evaluation Program for Fiscal Year 1979, 95th Cong., 2nd sess., 1978
(statement of Alton D. Slay, USAF Research and Development Deputy Chief of Staff), 344–58.
96 M. R. Wool, Final Summary Report Passive Nosetip Technology (PANT) Program, NTIS No. SAMSO-TR-75-250
(Mountain View, CA: Aerotherm Division/Acurex Corporation, 1975).
97 W. Burns J., ABRES Flight Test Evaluation of RV Accuracy, CDRL No. A023 (Redondo Beach, CA: TRW Systems
Group, 1974); and J. K. Cole et al., Test Report for SAMS Rain-Erosion Flights 3, 4, and 5, SLA-73-0565
(Albuquerque, NM: Sandia National Laboratories, 1973).
98 Office of Technology Assessment, MX Missile Basing (Washington, DC: Government Publishing Office,
1981), https://hdl.handle.net/2027/uc1.31210024829762; Stephen Weiner, “Systems and Technology,” in Ballistic Missile Defense, eds. Ashton Carter and Stephen Weiner (Washington, DC: Brookings Institution,
1984); Ruth Howes, “Hard Point Defenses,” in The Future of Land-Based Strategic Missiles, eds. Barbara G.
Levi, Mark Sakitt, and Art Hobson (New York: American Institute of Physics, 1989); Edward C. Burks,
“Reagan’s Decision on MX Is Reported,” New York Times, November 22, 1982, https://www.nytimes.
com/1982/11/22/us/reagan-s-decision-on-mx-is-reported.html; and Ashton Carter, “BMD Applications,”
in Ballistic Missile Defense, eds. Ashton Carter and Stephen Weiner (Washington, DC: Brookings Institution,
1984).
99 Mark Dubinskii et al., “Lasers for DEW Based on Fully Crystalline Fibers” (presentation, NDIA Army
Science & Technology Symposium and Showcase, Washington, DC, August 22, 2018), https://ndiastorage.
blob.core.usgovcloudapi.net/ndia/2018/armyst/Dubinskiy.pdf; Jeffrey Hecht, “High-Power Fiber
Lasers,” Optics and Photonics News, October 2018, https://www.osa-opn.org/home/articles/volume_29/
october_2018/features/high-power_fiber_lasers/; Jeffrey Hecht, “Fiber Lasers Mean Ray Guns Are
Coming,” IEEE Spectrum, March 27, 2018, https://spectrum.ieee.org/fiber-lasers-mean-ray-guns-arecoming;
Phillip Sprangle et al., “Incoherent Combining and Atmospheric Propagation of High-Power
Fiber Lasers for Directed-Energy Applications,” IEEE Journal of Quantum Electronics 45, no. 2 (February
2009), 138–48, doi:10.1109/JQE.2008.2002501; Barbara Gefvert et al., “Annual Laser Market Review &
Forecast 2019: What Goes Up…,” Laser Focus World, January 1, 2019, https://www.laserfocusworld.com/
lasers-sources/article/16556290/annual-laser-market-review-forecast-2019-what-goes-up; 88th Air Base
Wing Public Affairs, “Air Force Research Laboratory Completes Successful Shoot down of Air-Launched
Missiles,” Wright-Patterson Air Force Base, U.S. Air Force, May 3, 2019, https://www.wpafb.af.mil/News/
Article-Display/Article/1834836/air-force-research-laboratory-completes-successful-shoot-down-of-airlaunched-m/;
Mikayla Mast, “High Energy Laser Engineers Engage with West Point Cadets,” www.army.mil,
March 18, 2020, https://www.army.mil/article/233764/high_energy_laser_engineers_engage_with_west_
point_cadets; Kyle Mizokami, “The U.S. Army Plans To Field the Most Powerful Laser Weapon Yet,” Popular
Mechanics, August 7, 2019, https://www.popularmechanics.com/military/weapons/a28636854/powerfullaser-weapon/;
Bryan Ripple, “Enemy Drone Operators May Soon Face the Power of THOR,” WrightPatterson
Air Force Base, September 24, 2019, https://www.wpafb.af.mil/DesktopModules/ArticleCS/
Print.aspx?PortalId=60&ModuleId=8664&Article=1969142; and Sydney J. Jr. Freedberg, “Killing Cruise
Missiles: Pentagon To Test Rival Lasers,” Breaking Defense, December 2, 2019, https://breakingdefense.
com/2019/12/exclusive-three-ways-to-kill-cruise-missiles-pentagon-to-test-rival-lasers/.
100 Jason Kaneshiro, “Picatinny Engineers Set Phasers to ‘Fry’,” www.army.mil, June 22, 2012, https://www.
army.mil/article/82262/picatinny_engineers_set_phasers_to_fry.
101 Missile Defense Agency, Missile Defense Agency Request for Information for Pulsed Laser, Notice
No. MDA21DVRFI011 (Huntsville, AL: Missile Defense Agency, 2021), https://sam.gov/
opp/0f8abd642c9544e485469c3b5a162208/view?keywords=%22pulsed%20laser%22&sort=-
relevance&index=&is_active=true&page=1.
102 Barton et al., “Report of the American Physical Society Study Group on Boost-Phase Intercept
Systems for National Missile Defense”; Mitchell H. Fields et al., “Initial Results from the AdvancedConcepts
Laboratory for Adaptive Optics and Tracking,” Laser Weapons Technology 4034 (2000),
doi:10.1117/12.391862; Kimberley A. Clarke, “Performance Optimization Study of a Common Aero
Vehicle Using a Legendre Pseudospectral Method” (Master’s thesis, Massachusetts Institute of Technology,
2003), http://hdl.handle.net/1721.1/28284; Edward A. Cloutis, Michael J. Gaffey, and Thomas F. Moslow,
“Spectral Reflectance Properties of Carbon-Bearing Materials,” Icarus 107, no. 2 (February 1994), 276–87,
doi:10.1006/icar.1994.1023; and Jan Stupl and Götz Neuneck, “Assessment of Long Range Laser Weapon
Engagements: The Case of the Airborne Laser,” Science & Global Security 18, no. 1 (February 26, 2010), 1–60,
doi:10.1080/08929880903422034.
103 Some commentators argue lasers could disrupt the flow field of hypersonic flight. By inducing unexpected
heating in areas such as near control surfaces or inlets, a high-powered laser might induce boundary layer transition or other aerothermal phenomena. Such an approach would call for lasers and adaptive optical
systems capable of producing extremely fine beam qualities at the target, which could concentrate energy
on localized points on a hypersonic weapon’s surface. Considerable obstacles appear to remain for such
approaches.
104 Philip E. Nielsen, Effects of Directed Energy Weapons (Washington, DC: National Defense University Press,
1994), https://permanent.fdlp.gov/lps36040/Nielsen-EDEW.pdf.
105 Donald Shiffler, “Directed Energy and Base Defense” (presentation, NDIA Armament Systems Forum,
Fredricksburg, VA, June 5, 2019).
106 Bernard Nalty, USAF Ballistic Missile Programs 1964-1966, 80-CVAH(S)-D233 (Washington, DC: U.S. Air
Force Historical Division, 1967), https://www.hsdl.org/?view&did=485675.
107 U.S. Department of Justice, “Chinese National Sentenced to 40 Months in Prison for Conspiring to
Illegally Export Military- and Space-Grade Technology from the United States to China,” U.S. Department
of Justice, October 18, 2019, https://www.justice.gov/opa/pr/chinese-national-sentenced-40-monthsprison-conspiring-illegally-export-military-and-space;
United States Attorney’s Office (Eastern District of
Virginia), “Chinese Nationals Sentenced 24 Months for Illegally Attempting to Export Radiation-Hardened
Microchips to the PRC,” Offices of the United States Attorneys, September 30, 2011, https://www.justice.
gov/archive/usao/vae/news/2011/09/20110930Chinesenr.html; and White House, Administration Strategy
for Mitigating The Theft of U.S. Trade Secrets (Washington, DC: White House, 2013), https://www.justice.gov/
criminal-ccips/file/938321/download.
108 Defense Science Board, Report of Defense Science Board Task Force on Time Critical Conventional Strike from
Strategic Standoff (Washington, DC: U.S. Department of Defense, 2009), https://dsb.cto.mil/reports/2000s/
ADA498403.pdf.
109 VanHerck, “Rethinking Homeland Defense.”
110 Stephen Biddle and Ivan Oelrich, “Future Warfare in the Western Pacific: Chinese Antiaccess/Area Denial,
U.S. AirSea Battle, and Command of the Commons in East Asia,” International Security 41, no. 1 (July 2016),
7–48, doi:10.1162/ISEC_a_00249.
111 Thomas G Mahnken et al., Tightening the Chain: Implementing a Strategy of Maritime Pressure in the Western
Pacific (Washington, DC: Center for Strategic and Budgetary Assessments, 2019), https://csbaonline.
org/research/publications/implementing-a-strategy-of-maritime-pressure-in-the-western-pacific/
publication/1.
112 U.S. Army, Army Air and Missile Defense 2028 (Washington, DC: U.S. Department of Defense, 2019), https://
www.smdc.army.mil/Portals/38/Documents/Publications/Publications/SMDC_0120_AMD-BOOK_Finalv2.
pdf; and Joseph Buontempo and Joseph Ringer, “Airbase Defense Falls Between the Cracks,” Joint Force
Quarterly 97 (April 2020), https://ndupress.ndu.edu/Media/News/News-Article-View/Article/2106563/
airbase-defense-falls-between-the-cracks/.
113 Lina Davydova and Natalia Kima, “Все Гениальное Просто: Как Генерал-Полковник Евгений
Колибернов «обхитрил» Американский «Першинг 2» [Everything Ingenious Is Simple: How Colonel
General Yevgeny Kolibernov ‘Outsmarted’ the American Pershing 2],” Zvezda, December 4, 2019, https://
tvzvezda.ru/news/20191241348-rKDQX.html; Timothy L. Thomas, “Kosovo and the Current Myth of
Information Superiority,” U.S. Army War College Quarterly: Parameters 30, no. 1 (2000), https://press.
armywarcollege.edu/cgi/viewcontent.cgi?article=1967&context=parameters.
114 U.S. Department of Defense, Summary of the 2018 National Defense Strategy of the United States of America:
Sharpening the American Military’s Competitive Edge (Washington, DC: U.S. Department of Defense, 2018),
https://dod.defense.gov/Portals/1/Documents/pubs/2018-National-Defense-Strategy-Summary.pdf.
115 Sam Goldsmith, “U.S. Conventional Access Strategy: Denying China a Conventional First-Strike Capability,” Naval War College Review 72, no. 2 (2019), 35–65, https://digital-commons.usnwc.edu/cgi/
viewcontent.cgi?article=7972&context=nwc-review.
116 Teague, “SBIRS Transformational Capability.”
117 Karako and Rumbaugh, Distributed Defense.
118 National Research Council, High-Speed, Maneuvering Weapons: A Threat to America’s Global Vigilance, Reach,
and Power, Unclassified Summary (Washington, DC: National Academies Press, 2016), doi:10.17226/23667.
119 Michael White, “Ensuring Defensive Hypersonic Technology Superiority for the DoD” (speech, IDGA
Counter Hypersonic Weapons Summit, Online, July 23, 2020).
120 Ibid.
121 National Research Council, High-Speed, Maneuvering Weapons.
122 Ibid.
123 Joint Chiefs of Staff, Countering Air and Missile Threats, JP 3-01 (Washington, DC: Joint Chiefs of Staff,
2017), https://www.jcs.mil/Portals/36/Documents/Doctrine/pubs/jp3_01.pdf.
124 Don Baker and Michael Waint, “Countering Air and Missile Threats: A Combination of Counter Air and
Integrated Air and Missile Defense in Joint Publication 3-01,” Fires, April 2018.
125 Ibid. The 2017 edition of JP 3-01 consequently expanded STRATCOM’s planning and cross-AOR analysis
function to minimize operational seams related to endoatmospheric missile defense.
126 VanHerck, “Rethinking Homeland Defense.”
127 Ibid.
128 Andrew Feickert, The Unified Command Plan and Combatant Commands: Background and Issues for ongress,
CRS Report No. R42077 (Washington, DC: Congressional Research Service, 2013), https://sgp.fas.org/crs/
natsec/R42077.pdf; and Jesse A. Jr. Wilson, “Have Adversary Missiles Become a Revolution in Military
Affairs?,” Air and Space Power Journal 8, no. 6 (2014), 99–101.
129 Thomas Roberts, “Space Launch to Low Earth Orbit: How Much Does It Cost?,” CSIS, Aerospace Security,
September 2, 2020, https://aerospace.csis.org/data/space-launch-to-low-earth-orbit-how-much-does-itcost/;
Stockdale and Aughenbaugh, “Low-Cost Access to Space: Military Opportunities and Challenges”;
Joseph Trevithick, “Work Begins On Starlink-Like Constellation Of Small Hypersonic Missile-Tracking
Satellites,” The Drive, October 6, 2020, https://www.thedrive.com/the-war-zone/36909/work-begins-onstarlink-like-constellation-of-small-hypersonic-missile-tracking-satellites;
Theresa Hitchens, “Under
Senate’s Eye, NRO, NGA Stress Commercial Imagery Plans,” Breaking Defense, July 9, 2020, https://
breakingdefense.sites.breakingmedia.com/2020/07/under-senates-eye-nro-nga-stress-commercialimagery-plans/;
C. Todd Lopez, “National Geospatial-Intelligence Agency in Midst of Revolution,” U.S.
Department of Defense, accessed September 1, 2021, https://www.defense.gov/Explore/News/Article/
Article/2447871/national-geospatial-intelligence-agency-in-midst-of-revolution/; and Air Force Space
Command, The Future of Space 2060 and Implications for U.S. Strategy: Report on the Space Futures Workshop.
130 Donald L. Schmidt, Carbon-Carbon Composites (CCC) – A Historical Perspective, WL-TR-96-4107 (Dayton,
OH: University of Dayton Research Institute, 1996), https://apps.dtic.mil/sti/citations/ADA325314; Air
Force Research Laboratory, Manufacturing of Carbon-Carbon (C-C) Composites for Hypersonic Applications
(MOC3HA) Statement of Objectives, Notice No. MDA21DVRFI011 (Washington, DC: U.S. Air Force, 2019),
3, https://govtribe.com/opportunity/federal-contract-opportunity/manufacturing-of-carbon-carboncomposites-for-hypersonic-applications-moc3ha-fa865020s5004;
and Vernon Bechel, “Tape-Wrapped
‘Aeroshells’ Cut Cost of Hypersonic Glide Vehicles,” Wright-Patterson Air Force Base, U.S. Air Force,
October 19, 2012, http://www.wpafb.af.mil/News/Article-Display/Article/399513/tape-wrappedaeroshells-cut-cost-of-hypersonic-glide-vehicles.
31 Amanda Macias, “Russia Will Only Make a Few Units of a Hypersonic Weapon Putin Bragged about, US
Intelligence Says,” CNBC, July 1, 2019, https://www.cnbc.com/2019/07/01/russia-will-make-few-units-ofhypersonic-weapon-putin-bragged-about.html.
132 Dave Kuntz et al., “Ablation Modeling Capabilities and Development Efforts at Sandia National
Laboratories” (presentation, 7th Ablation Workshop, Tullahoma, TN, October 21, 2015); and Cummings
et al., “Advancing Challenging Aerothermodynamic and Propulsion System Phenomena via Hypersonic
Vehicle Simulation.”
133 Timothy D. West, “U.S. Air Force Investments in Hypersonic Test Technologies & Infrastructure”
(presentation, NDIA 33rd Annual National Test & Evaluation Conference, Solomons, MD, May 16, 2018),
https://ndia.dtic.mil/2017/2017test.html; and John D. Schmisseur, “Hypersonics Into the 21st Century:
A Perspective on AFOSR-Sponsored Research in Aerothermodynamics,” in 43rd Fluid Dynamics Conference
(San Diego, CA: American Institute of Aeronautics and Astronautics, 2013), doi:10.2514/6.2013-2606.
134 John D. Schmisseur, “Efforts to Guide Hypersonic Workforce Development in Support of Defensive
Activities” (presentation, IDGA Counter Hypersonic Weapons Summit, Online, July 24, 2020).
135 Aaron Mehta, “US Army to Test Hypersonic Weapons on This College Campus,” Defense News, May
14, 2020, https://www.defensenews.com/battlefield-tech/2020/05/14/hypersonic-weapons-testfacility-approved-by-texas-am/;
and Texas A&M University System, “Texas A&M System To Lead $100
Million Hypersonic Research Consortium,” Texas A&M Today, October 26, 2020, https://today.tamu.
edu/2020/10/26/texas-am-system-to-lead-consortium-on-advancing-hypersonic-flight-systems/.
136 Sylvia Johnson et al., “Recent Developments in Ultra High Temperature Ceramics at NASA Ames,” in 16th
AIAA/DLR/DGLR International Space Planes and Hypersonic Systems and Technologies Conference (16th AIAA/
DLR/DGLR International Space Planes and Hypersonic Systems and Technologies Conference, Bremen,
Germany: American Institute of Aeronautics and Astronautics, 2009), doi:10.2514/6.2009-7219; and
Marineau, “ONR Foundational Research Supporting Defensive Hypersonic Efforts.”
137 Craig A. Stephens, “Overview of NASA’s Hypersonic Air-Breathing Materials & Structures Discipline”
(presentation, NASA Technical Conference, Cleveland, OH, March 13, 2012); Glass, “Hypersonic Materials
and Structures”; and A. Paul et al., “UHTC Composites for Hypersonic Applications,” American Ceramic
Society Bulletin 91, no. 1 (February 2012), 22–29B, http://ceramics.org/wp-content/uploads/2012/01/
janfeb12cover.pdf.
138 T. A. Jackson, “HIFiRE (Hypersonic International Flight Research and Experimentation): Affordable
Hypersonic Flight Experimentation” (presentation, Wright-Patterson Air Force Base, OH, September 14,
2007); Kevin Jackson, Mark Gruber, and Salvatore Buccellato, “HIFiRE Flight 2 Project Overview and Status
Update 2011,” in 17th AIAA International Space Planes and Hypersonic Systems and Technologies Conference
(17th AIAA International Space Planes and Hypersonic Systems and Technologies Conference, San
Francisco, California: American Institute of Aeronautics and Astronautics, 2011), doi:10.2514/6.2011-
2202; Scott Berry, Roger Kimmel, and Eli Reshotko, “Recommendations for Hypersonic Boundary
Layer Transition Flight Testing,” in 41st AIAA Fluid Dynamics Conference and Exhibit (41st AIAA
Fluid Dynamics Conference and Exhibit, Honolulu, Hawaii: American Institute of Aeronautics and
Astronautics, 2011), doi:10.2514/6.2011-3415; Guy Norris, “Model Program: International Hypersonic
Research Effort Sets up for Fast-Paced Test Plan,” Aviation Week and Space Technology, November 26,
2012; Schmisseur, “Hypersonics Into the 21st Century: A Perspective on AFOSR-Sponsored Research
in Aerothermodynamics”; and Garry A. Haase, “Guiding AFRL Munitions Efforts to Support a USAF
Hypersonic Effort” (IDGA Hypersonic Weapons Summit, Online, April 28, 2021).
This report is made possible by support from Raytheon Technologies and Lockheed Martin, and by general support to CSIS.DOWNLOADS Download the Full Report (2.7 MB)
Credits | CSIS
[…] and Moscow’s superweapons—which have leaped ahead of the Pentagon’s arsenal—on the cheap. That’s according to a new report from the Center for Strategic and International Studies (CSIS), a Washington think tank. It calls on the Biden administration to roll out a new playbook for […]
[…] war in Ukraine and its implication to the overall arms race with the US and China. Also read a comprehensive report on hypersonic weapon system being developed by the US published by GS on February 8, […]